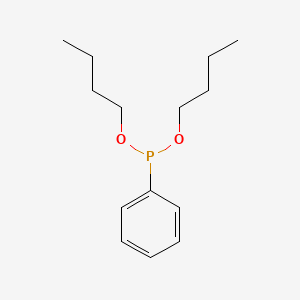
Dibutyl phenylphosphonite
Overview
Description
Dibutyl phenylphosphonite is an organophosphorus compound with the chemical formula C16H25O2P. It is a colorless to pale yellow liquid that is used in various chemical reactions and industrial applications. This compound is known for its role as a stabilizer and antioxidant in polymers and other materials.
Preparation Methods
Synthetic Routes and Reaction Conditions
Dibutyl phenylphosphonite can be synthesized through the reaction of phenylphosphonic dichloride with butanol in the presence of a base. The reaction typically occurs under reflux conditions, and the product is purified through distillation. The general reaction is as follows:
PhPCl2+2BuOH→PhP(OBu)2+2HCl
Industrial Production Methods
In industrial settings, this compound is produced using continuous flow reactors to ensure consistent quality and yield. The process involves the controlled addition of reactants and the use of catalysts to enhance the reaction rate. The product is then subjected to purification steps such as distillation and filtration to remove impurities.
Chemical Reactions Analysis
Types of Reactions
Dibutyl phenylphosphonite undergoes various chemical reactions, including:
Oxidation: It can be oxidized to form dibutyl phenylphosphonate.
Substitution: It can participate in nucleophilic substitution reactions where the butyl groups are replaced by other nucleophiles.
Hydrolysis: It can be hydrolyzed to form phenylphosphonic acid and butanol.
Common Reagents and Conditions
Oxidation: Common oxidizing agents include hydrogen peroxide and oxygen. The reaction is typically carried out at elevated temperatures.
Substitution: Reagents such as alkyl halides or alcohols can be used in the presence of a base to facilitate substitution reactions.
Hydrolysis: Acidic or basic conditions can be used to hydrolyze this compound.
Major Products Formed
Oxidation: Dibutyl phenylphosphonate.
Substitution: Various substituted phosphonites depending on the nucleophile used.
Hydrolysis: Phenylphosphonic acid and butanol.
Scientific Research Applications
Catalytic Applications
Dibutyl phenylphosphonite is primarily recognized for its utility as a ligand in coordination chemistry and catalysis. Its phosphorus center can coordinate with transition metals, facilitating various chemical reactions.
- Homogeneous Catalysis : DBPP is utilized in liquid-liquid biphasic systems, enhancing reaction efficiency by providing a stable environment for catalytic processes. Its ability to form complexes with metals like palladium and platinum allows it to participate in hydrophosphination reactions, which are crucial for synthesizing organophosphorus compounds .
- Mechanistic Studies : Research indicates that DBPP can act as a catalyst in the phosphorylation of alcohols and phenols, leading to the formation of phosphonates and phosphonites. This application is significant in the synthesis of biologically active molecules .
Material Science
DBPP has applications in the development of advanced materials, particularly in coatings and polymers.
- Stabilizers in Polymers : The compound serves as a stabilizer for polyolefins and other polymers, enhancing their thermal stability and resistance to oxidative degradation. This property is vital for extending the lifespan of materials used in various industrial applications .
- Mechanoluminescent Materials : Recent studies have explored the incorporation of DBPP into mechanoluminescent materials, which emit light when mechanically stressed. This application has potential uses in safety devices and smart materials that respond to physical stimuli .
Biological Applications
DBPP's biological activity makes it a candidate for various applications in medicinal chemistry.
- Antioxidant Properties : Research has shown that this compound exhibits antioxidant properties, which can protect cells from oxidative stress. This characteristic is particularly relevant in developing therapeutic agents aimed at combating diseases related to oxidative damage .
- Drug Development : Its role as a synthetic intermediate allows for the creation of phosphorus-containing drugs, which are essential in treating various conditions, including cancer and neurodegenerative diseases. DBPP can be modified to enhance its pharmacological properties, making it a versatile compound in drug design .
Case Study 1: Catalytic Efficiency
A study highlighted the effectiveness of DBPP as a ligand in palladium-catalyzed reactions. The research demonstrated that using DBPP significantly increased the yield of desired products compared to traditional ligands. The mechanism involved coordination with palladium, facilitating the activation of substrates under mild conditions .
Case Study 2: Material Development
In another study, DBPP was incorporated into polymer matrices to improve their mechanical properties. The results indicated that polymers containing DBPP exhibited enhanced toughness and resistance to thermal degradation, showcasing its potential for use in high-performance materials .
Mechanism of Action
The mechanism of action of dibutyl phenylphosphonite involves its ability to donate electrons and neutralize free radicals, thereby acting as an antioxidant. It interacts with molecular targets such as reactive oxygen species (ROS) and prevents oxidative damage to cells and materials. The pathways involved include the scavenging of free radicals and the inhibition of oxidative chain reactions.
Comparison with Similar Compounds
Similar Compounds
Dibutyl phosphite: Similar in structure but lacks the phenyl group.
Triphenyl phosphite: Contains three phenyl groups instead of butyl groups.
Dibutyl phenylphosphonate: The oxidized form of dibutyl phenylphosphonite.
Uniqueness
This compound is unique due to its combination of butyl and phenyl groups, which provide a balance of hydrophobic and hydrophilic properties. This makes it an effective stabilizer and antioxidant in various applications. Its ability to undergo multiple types of chemical reactions also adds to its versatility in research and industrial settings.
Biological Activity
Dibutyl phenylphosphonite (DBPP) is an organophosphorus compound that has garnered attention for its biological activity, particularly in the context of its potential therapeutic applications and mechanisms of action. This article aims to consolidate existing research findings, case studies, and data tables to provide a comprehensive overview of the biological activities associated with DBPP.
- Chemical Formula : CHOP
- Molecular Weight : 250.30 g/mol
- Structure : DBPP consists of a phenyl group attached to a phosphonite moiety with two butyl groups.
Antimicrobial Properties
DBPP has shown significant antimicrobial activity, particularly against various strains of bacteria and fungi. Studies have indicated that DBPP can disrupt microbial cell membranes, leading to cell lysis and death. The mechanism appears to involve the interaction of the phosphonite group with lipid bilayers, which alters membrane integrity.
Enzyme Inhibition
Research has demonstrated that DBPP acts as an inhibitor for several key enzymes:
- Glycogen Synthase Kinase 3 Beta (GSK-3β) : DBPP exhibits a moderate inhibitory effect on GSK-3β, which is implicated in various cellular processes including metabolism and cell survival. Molecular docking studies suggest that DBPP binds preferentially to the substrate-binding site of GSK-3β, leading to increased ATP accumulation in enzymatic assays (IC = 2.0 µM) .
- Plasmodium falciparum : Preliminary studies indicate that DBPP may possess antimalarial properties through mechanisms yet to be fully elucidated. Its interaction with Plasmodium falciparum has been noted, although resistance studies have not yielded definitive insights into its mode of action against this pathogen .
Cytotoxicity
In vitro studies have assessed the cytotoxic effects of DBPP on various human cell lines. Results showed minimal cytotoxicity at therapeutic concentrations, indicating a favorable safety profile for potential pharmaceutical applications .
Case Studies
- Antimicrobial Efficacy : A study conducted on the efficacy of DBPP against Staphylococcus aureus demonstrated a significant reduction in bacterial viability at concentrations as low as 50 µg/mL. The study highlighted the compound's potential as a biocide in clinical settings.
- GSK-3β Inhibition : In a controlled enzymatic assay, DBPP was tested alongside other known inhibitors of GSK-3β. The results indicated that DBPP's mixed inhibition mechanism could be exploited for therapeutic interventions in conditions such as diabetes and neurodegenerative diseases .
Table 1: Summary of Biological Activities of this compound
Table 2: Molecular Docking Results for GSK-3β Interaction
Binding Site | Binding Affinity (kcal/mol) | Interaction Type |
---|---|---|
Substrate-binding site | -6.9 | Hydrogen bonds |
ATP-binding site | -6.1 | Steric interactions |
Properties
IUPAC Name |
dibutoxy(phenyl)phosphane | |
---|---|---|
Details | Computed by Lexichem TK 2.7.0 (PubChem release 2021.05.07) | |
Source | PubChem | |
URL | https://pubchem.ncbi.nlm.nih.gov | |
Description | Data deposited in or computed by PubChem | |
InChI |
InChI=1S/C14H23O2P/c1-3-5-12-15-17(16-13-6-4-2)14-10-8-7-9-11-14/h7-11H,3-6,12-13H2,1-2H3 | |
Details | Computed by InChI 1.0.6 (PubChem release 2021.05.07) | |
Source | PubChem | |
URL | https://pubchem.ncbi.nlm.nih.gov | |
Description | Data deposited in or computed by PubChem | |
InChI Key |
YBAAIJIDIRQVLY-UHFFFAOYSA-N | |
Details | Computed by InChI 1.0.6 (PubChem release 2021.05.07) | |
Source | PubChem | |
URL | https://pubchem.ncbi.nlm.nih.gov | |
Description | Data deposited in or computed by PubChem | |
Canonical SMILES |
CCCCOP(C1=CC=CC=C1)OCCCC | |
Details | Computed by OEChem 2.3.0 (PubChem release 2021.05.07) | |
Source | PubChem | |
URL | https://pubchem.ncbi.nlm.nih.gov | |
Description | Data deposited in or computed by PubChem | |
Molecular Formula |
C14H23O2P | |
Details | Computed by PubChem 2.1 (PubChem release 2021.05.07) | |
Source | PubChem | |
URL | https://pubchem.ncbi.nlm.nih.gov | |
Description | Data deposited in or computed by PubChem | |
Molecular Weight |
254.30 g/mol | |
Details | Computed by PubChem 2.1 (PubChem release 2021.05.07) | |
Source | PubChem | |
URL | https://pubchem.ncbi.nlm.nih.gov | |
Description | Data deposited in or computed by PubChem | |
Synthesis routes and methods
Procedure details
Retrosynthesis Analysis
AI-Powered Synthesis Planning: Our tool employs the Template_relevance Pistachio, Template_relevance Bkms_metabolic, Template_relevance Pistachio_ringbreaker, Template_relevance Reaxys, Template_relevance Reaxys_biocatalysis model, leveraging a vast database of chemical reactions to predict feasible synthetic routes.
One-Step Synthesis Focus: Specifically designed for one-step synthesis, it provides concise and direct routes for your target compounds, streamlining the synthesis process.
Accurate Predictions: Utilizing the extensive PISTACHIO, BKMS_METABOLIC, PISTACHIO_RINGBREAKER, REAXYS, REAXYS_BIOCATALYSIS database, our tool offers high-accuracy predictions, reflecting the latest in chemical research and data.
Strategy Settings
Precursor scoring | Relevance Heuristic |
---|---|
Min. plausibility | 0.01 |
Model | Template_relevance |
Template Set | Pistachio/Bkms_metabolic/Pistachio_ringbreaker/Reaxys/Reaxys_biocatalysis |
Top-N result to add to graph | 6 |
Feasible Synthetic Routes
Disclaimer and Information on In-Vitro Research Products
Please be aware that all articles and product information presented on BenchChem are intended solely for informational purposes. The products available for purchase on BenchChem are specifically designed for in-vitro studies, which are conducted outside of living organisms. In-vitro studies, derived from the Latin term "in glass," involve experiments performed in controlled laboratory settings using cells or tissues. It is important to note that these products are not categorized as medicines or drugs, and they have not received approval from the FDA for the prevention, treatment, or cure of any medical condition, ailment, or disease. We must emphasize that any form of bodily introduction of these products into humans or animals is strictly prohibited by law. It is essential to adhere to these guidelines to ensure compliance with legal and ethical standards in research and experimentation.