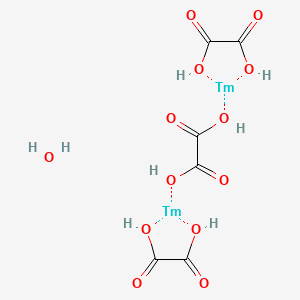
Oxalic acid;thulium;hydrate
Overview
Description
Oxalic acid;thulium;hydrate is a compound that combines oxalic acid, thulium, and water molecules. Thulium is a rare earth element, and when combined with oxalic acid and water, it forms a hydrated complex
Mechanism of Action
Target of Action
Oxalic acid, a strong dicarboxylic acid, is found in many plants and vegetables . It is produced in the body by metabolism of glyoxylic acid or ascorbic acid . Thulium(III) oxalate is the oxalate of thulium with the chemical formula Tm2(C2O4)3
Mode of Action
Oxalic acid is known to have good chelating properties with divalent ions like manganese or ferric ions . This suggests that it may interact with its targets through chelation, potentially influencing the bioavailability of these ions.
Biochemical Pathways
Oxalic acid and oxalates are secondary metabolites secreted to the surrounding environment by fungi, bacteria, and plants . They are involved indirectly in many processes, such as pollutant bioremediation, wood degradation, biodeterioration processes, and rock weathering processes . Due to their chelating ability, oxalates are considered as good factors in the detoxification of heavy metals, including aluminium, lead, copper, and cadmium ions .
Action Environment
Environmental factors can influence the action, efficacy, and stability of oxalic acid; thulium; hydrate. For instance, the secretion of oxalic acid from plant roots can easily reduce Tl(III) to Tl(I), and more than 80% of Tl(III) could still be remained in the system by controlling the treatment time and the number of plant roots to decrease the amount of oxalic acid secreted by roots . This suggests that environmental conditions, such as the presence of other ions and the pH of the environment, may influence the action of this compound.
Biochemical Analysis
Biochemical Properties
Oxalic acid is a widespread organic acid found in various organisms, including plants, animals, bacteria, and fungi . It plays a role in several biochemical reactions, often acting as a chelating agent with divalent ions like manganese or ferric ions . Thulium, on the other hand, is less well-studied in biological contexts, but its interactions with oxalic acid could potentially influence various enzymes, proteins, and other biomolecules.
Cellular Effects
The effects of oxalic acid thulium hydrate on cellular processes are not well-documented. Oxalic acid has been shown to influence cell function in various ways. For instance, it can affect the pH of the cellular environment, potentially influencing cell signaling pathways and metabolic processes
Molecular Mechanism
Oxalic acid can interact with various biomolecules, potentially leading to changes in gene expression or enzyme activity . The role of thulium in these processes is less clear, but it may influence the binding interactions of oxalic acid with other molecules.
Temporal Effects in Laboratory Settings
The stability and degradation of oxalic acid thulium hydrate over time in laboratory settings have not been extensively studied. Oxalic acid is known to be involved in various processes such as pollutant bioremediation, wood degradation, and rock weathering processes
Metabolic Pathways
Oxalic acid is involved in several metabolic pathways, interacting with various enzymes and cofactors . It is also linked to nutrient availability and mineral weathering in soil . The metabolic pathways involving thulium are less well-known, and the interaction of thulium with oxalic acid could potentially influence these pathways.
Transport and Distribution
Oxalic acid is known to be secreted into the environment by various organisms , and it could potentially be transported within cells via similar mechanisms. The role of thulium in these processes is unclear.
Subcellular Localization
Oxalic acid is a small, soluble molecule that could potentially localize to various compartments within the cell . The presence of thulium could influence this localization, potentially directing the compound to specific organelles or compartments.
Preparation Methods
Synthetic Routes and Reaction Conditions
The preparation of oxalic acid;thulium;hydrate typically involves the reaction of thulium salts with oxalic acid in an aqueous solution. The reaction can be represented as follows: [ \text{Tm}^{3+} + 3 \text{H}_2\text{C}_2\text{O}_4 \rightarrow \text{Tm}_2(\text{C}_2\text{O}_4)_3 + 6 \text{H}^+ ]
This reaction is usually carried out under acidic conditions to ensure the complete dissolution of thulium salts and the formation of the hydrated complex .
Industrial Production Methods
Industrial production of this compound involves the controlled mixing of thulium salts and oxalic acid in large-scale reactors. The reaction mixture is then filtered to remove any impurities, and the resulting product is crystallized to obtain the pure hydrated complex. The crystallization process is typically carried out at low temperatures to ensure the formation of well-defined crystals.
Chemical Reactions Analysis
Types of Reactions
Oxalic acid;thulium;hydrate undergoes various chemical reactions, including:
Oxidation: Oxalic acid can be oxidized to carbon dioxide and water in the presence of strong oxidizing agents.
Reduction: Thulium ions can be reduced to their metallic state under specific conditions.
Substitution: The oxalate ions in the complex can be substituted with other ligands in the presence of suitable reagents.
Common Reagents and Conditions
Oxidizing Agents: Potassium permanganate (KMnO4) and hydrogen peroxide (H2O2) are commonly used oxidizing agents for oxalic acid.
Reducing Agents: Sodium borohydride (NaBH4) and lithium aluminum hydride (LiAlH4) can be used to reduce thulium ions.
Substitution Reactions: Ligands such as ethylenediaminetetraacetic acid (EDTA) can be used to substitute oxalate ions in the complex.
Major Products
Oxidation: Carbon dioxide (CO2) and water (H2O).
Reduction: Metallic thulium ™.
Substitution: Complexes with different ligands, such as thulium-EDTA complexes.
Scientific Research Applications
Oxalic acid;thulium;hydrate has several scientific research applications, including:
Biology: Investigated for its potential use in biological imaging and as a contrast agent in magnetic resonance imaging (MRI).
Medicine: Studied for its potential therapeutic applications, including its use in targeted drug delivery systems.
Industry: Used in the production of specialized materials and as a component in certain industrial processes.
Comparison with Similar Compounds
Similar Compounds
Oxalic acid;erbium;hydrate: Similar in structure but contains erbium instead of thulium.
Oxalic acid;ytterbium;hydrate: Contains ytterbium and has similar chemical properties.
Oxalic acid;lutetium;hydrate: Contains lutetium and is used in similar applications.
Uniqueness
Oxalic acid;thulium;hydrate is unique due to the specific properties of thulium, which include its magnetic and luminescent characteristics. These properties make it particularly useful in applications such as MRI contrast agents and luminescent materials .
Properties
IUPAC Name |
oxalic acid;thulium;hydrate | |
---|---|---|
Details | Computed by Lexichem TK 2.7.0 (PubChem release 2021.05.07) | |
Source | PubChem | |
URL | https://pubchem.ncbi.nlm.nih.gov | |
Description | Data deposited in or computed by PubChem | |
InChI |
InChI=1S/3C2H2O4.H2O.2Tm/c3*3-1(4)2(5)6;;;/h3*(H,3,4)(H,5,6);1H2;; | |
Details | Computed by InChI 1.0.6 (PubChem release 2021.05.07) | |
Source | PubChem | |
URL | https://pubchem.ncbi.nlm.nih.gov | |
Description | Data deposited in or computed by PubChem | |
InChI Key |
ALOPYZRFVDVKRS-UHFFFAOYSA-N | |
Details | Computed by InChI 1.0.6 (PubChem release 2021.05.07) | |
Source | PubChem | |
URL | https://pubchem.ncbi.nlm.nih.gov | |
Description | Data deposited in or computed by PubChem | |
Canonical SMILES |
C(=O)(C(=O)O)O.C(=O)(C(=O)O)O.C(=O)(C(=O)O)O.O.[Tm].[Tm] | |
Details | Computed by OEChem 2.3.0 (PubChem release 2021.05.07) | |
Source | PubChem | |
URL | https://pubchem.ncbi.nlm.nih.gov | |
Description | Data deposited in or computed by PubChem | |
Molecular Formula |
C6H8O13Tm2 | |
Details | Computed by PubChem 2.1 (PubChem release 2021.05.07) | |
Source | PubChem | |
URL | https://pubchem.ncbi.nlm.nih.gov | |
Description | Data deposited in or computed by PubChem | |
Molecular Weight |
625.99 g/mol | |
Details | Computed by PubChem 2.1 (PubChem release 2021.05.07) | |
Source | PubChem | |
URL | https://pubchem.ncbi.nlm.nih.gov | |
Description | Data deposited in or computed by PubChem | |
Retrosynthesis Analysis
AI-Powered Synthesis Planning: Our tool employs the Template_relevance Pistachio, Template_relevance Bkms_metabolic, Template_relevance Pistachio_ringbreaker, Template_relevance Reaxys, Template_relevance Reaxys_biocatalysis model, leveraging a vast database of chemical reactions to predict feasible synthetic routes.
One-Step Synthesis Focus: Specifically designed for one-step synthesis, it provides concise and direct routes for your target compounds, streamlining the synthesis process.
Accurate Predictions: Utilizing the extensive PISTACHIO, BKMS_METABOLIC, PISTACHIO_RINGBREAKER, REAXYS, REAXYS_BIOCATALYSIS database, our tool offers high-accuracy predictions, reflecting the latest in chemical research and data.
Strategy Settings
Precursor scoring | Relevance Heuristic |
---|---|
Min. plausibility | 0.01 |
Model | Template_relevance |
Template Set | Pistachio/Bkms_metabolic/Pistachio_ringbreaker/Reaxys/Reaxys_biocatalysis |
Top-N result to add to graph | 6 |
Feasible Synthetic Routes
Disclaimer and Information on In-Vitro Research Products
Please be aware that all articles and product information presented on BenchChem are intended solely for informational purposes. The products available for purchase on BenchChem are specifically designed for in-vitro studies, which are conducted outside of living organisms. In-vitro studies, derived from the Latin term "in glass," involve experiments performed in controlled laboratory settings using cells or tissues. It is important to note that these products are not categorized as medicines or drugs, and they have not received approval from the FDA for the prevention, treatment, or cure of any medical condition, ailment, or disease. We must emphasize that any form of bodily introduction of these products into humans or animals is strictly prohibited by law. It is essential to adhere to these guidelines to ensure compliance with legal and ethical standards in research and experimentation.