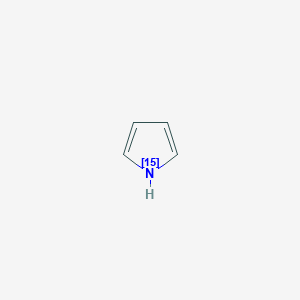
Pyrrole-15N
Overview
Description
Pyrrole is a heterocyclic, aromatic, organic compound, a five-membered ring with the formula C4H4NH . It is a colorless volatile liquid that darkens readily upon exposure to air . Substituted derivatives are also called pyrroles .
Synthesis Analysis
A method was developed for the synthesis of selectively labeled [15N]indole, allowing to obtain the target product with high chemical and isotopic purity . The Paal-Knorr pyrrole condensation of 2,5-dimethoxytetrahydrofuran with various amines and sulfonamines in water in the presence of a catalytic amount of iron(III) chloride allows the synthesis of N-substituted pyrroles under very mild reaction conditions in good to excellent yields .Molecular Structure Analysis
Pyrrole is a 5-membered aromatic heterocycle, like furan and thiophene . Unlike furan and thiophene, it has a dipole in which the positive end lies on the side of the heteroatom, with a dipole moment of 1.58 D . The chemical structure of polypyrrole (PPy) has been studied by 15N CPMAS NMR experiments on the 15N-labeled material .Chemical Reactions Analysis
Pyrrole undergoes electrophilic substitution reactions at carbon-2 and gives 2-substituted product . The reaction of the pyrrole with the conjugated acids of ketones, or aldehydes, gives rise to an alcohol which rapidly dehydrates to give a carbonium ion which is stabilized by resonance .Physical And Chemical Properties Analysis
Pyrrole is a colorless liquid, with a boiling point of 131° C . It readily turns into a brown color on air exposure, is sparingly soluble in water but dissolves in ethanol and ether . Its odor is similar to chloroform . Pyrrole is a very weak base with a pKa of about −3.8 and its protonation results in the loss of aromatic property .Scientific Research Applications
Understanding Extraterrestrial Organic Matter
Research on insoluble organic matter (IOM) from meteorites like Orgueil and Murchison utilized Pyrrole-15N to study the macromolecular structure. Techniques such as pyrolysis and solid state 15N nuclear magnetic resonance (NMR) helped identify nitrogen sequestered in heterocyclic aromatic rings, including pyrrole. This study contributes to our understanding of the origin and formation of amino acids in space, suggesting they might be products of planetesimal subsurface chemistry rather than hydrolysis of IOM or from a common precursor (Remusat et al., 2005).
Organic Geochemistry and Spectroscopy
15N labeled pyrroles, synthesized from commercially available 15N glycine or sodium nitrite, were utilized to prepare per-15N labeled porphyrins. These labeled compounds are essential for analyzing and assigning vibrational spectra to sedimentary porphyrins, advancing our understanding in organic geochemistry and spectroscopy (Lash & Chen, 2005).
Advances in Pyrrole Synthesis
The synthesis of pyrroles, crucial for various scientific fields including biology and materials science, has seen significant advancements. Multicomponent reactions provide an efficient and environmentally friendly method for creating substituted and functionalized pyrrole derivatives, highlighting the versatility of Pyrrole-15N in synthetic chemistry (Estévez et al., 2014).
Environmental and Material Applications
Research on nanocomposites involving polypyrrole and mesoporous silica SBA-15 explored the adsorption behaviors of dyes from aqueous solutions, indicating Pyrrole-15N's potential in environmental remediation and material science applications (Boukoussa et al., 2018).
Characterizing Nitrogen in Carbonaceous Solids
The combination of XPS and solid-state 15N NMR was employed to characterize nitrogen forms in carbonaceous materials, providing insights into the roles of pyridinic and pyrrolic nitrogen in such compounds. This research has implications for understanding nitrogen-doped carbon materials in various applications, including catalysis and energy storage (Kelemen et al., 2002).
Mechanism of Action
Safety and Hazards
properties
IUPAC Name |
(115N)1H-pyrrole | |
---|---|---|
Details | Computed by LexiChem 2.6.6 (PubChem release 2019.06.18) | |
Source | PubChem | |
URL | https://pubchem.ncbi.nlm.nih.gov | |
Description | Data deposited in or computed by PubChem | |
InChI |
InChI=1S/C4H5N/c1-2-4-5-3-1/h1-5H/i5+1 | |
Details | Computed by InChI 1.0.5 (PubChem release 2019.06.18) | |
Source | PubChem | |
URL | https://pubchem.ncbi.nlm.nih.gov | |
Description | Data deposited in or computed by PubChem | |
InChI Key |
KAESVJOAVNADME-HOSYLAQJSA-N | |
Details | Computed by InChI 1.0.5 (PubChem release 2019.06.18) | |
Source | PubChem | |
URL | https://pubchem.ncbi.nlm.nih.gov | |
Description | Data deposited in or computed by PubChem | |
Canonical SMILES |
C1=CNC=C1 | |
Details | Computed by OEChem 2.1.5 (PubChem release 2019.06.18) | |
Source | PubChem | |
URL | https://pubchem.ncbi.nlm.nih.gov | |
Description | Data deposited in or computed by PubChem | |
Isomeric SMILES |
C1=C[15NH]C=C1 | |
Details | Computed by OEChem 2.1.5 (PubChem release 2019.06.18) | |
Source | PubChem | |
URL | https://pubchem.ncbi.nlm.nih.gov | |
Description | Data deposited in or computed by PubChem | |
Molecular Formula |
C4H5N | |
Details | Computed by PubChem 2.1 (PubChem release 2019.06.18) | |
Source | PubChem | |
URL | https://pubchem.ncbi.nlm.nih.gov | |
Description | Data deposited in or computed by PubChem | |
Molecular Weight |
68.08 g/mol | |
Details | Computed by PubChem 2.1 (PubChem release 2021.05.07) | |
Source | PubChem | |
URL | https://pubchem.ncbi.nlm.nih.gov | |
Description | Data deposited in or computed by PubChem | |
Product Name |
CID 21766225 |
Synthesis routes and methods I
Procedure details
Synthesis routes and methods II
Procedure details
Synthesis routes and methods III
Procedure details
Synthesis routes and methods IV
Procedure details
Synthesis routes and methods V
Procedure details
Retrosynthesis Analysis
AI-Powered Synthesis Planning: Our tool employs the Template_relevance Pistachio, Template_relevance Bkms_metabolic, Template_relevance Pistachio_ringbreaker, Template_relevance Reaxys, Template_relevance Reaxys_biocatalysis model, leveraging a vast database of chemical reactions to predict feasible synthetic routes.
One-Step Synthesis Focus: Specifically designed for one-step synthesis, it provides concise and direct routes for your target compounds, streamlining the synthesis process.
Accurate Predictions: Utilizing the extensive PISTACHIO, BKMS_METABOLIC, PISTACHIO_RINGBREAKER, REAXYS, REAXYS_BIOCATALYSIS database, our tool offers high-accuracy predictions, reflecting the latest in chemical research and data.
Strategy Settings
Precursor scoring | Relevance Heuristic |
---|---|
Min. plausibility | 0.01 |
Model | Template_relevance |
Template Set | Pistachio/Bkms_metabolic/Pistachio_ringbreaker/Reaxys/Reaxys_biocatalysis |
Top-N result to add to graph | 6 |
Feasible Synthetic Routes
Disclaimer and Information on In-Vitro Research Products
Please be aware that all articles and product information presented on BenchChem are intended solely for informational purposes. The products available for purchase on BenchChem are specifically designed for in-vitro studies, which are conducted outside of living organisms. In-vitro studies, derived from the Latin term "in glass," involve experiments performed in controlled laboratory settings using cells or tissues. It is important to note that these products are not categorized as medicines or drugs, and they have not received approval from the FDA for the prevention, treatment, or cure of any medical condition, ailment, or disease. We must emphasize that any form of bodily introduction of these products into humans or animals is strictly prohibited by law. It is essential to adhere to these guidelines to ensure compliance with legal and ethical standards in research and experimentation.