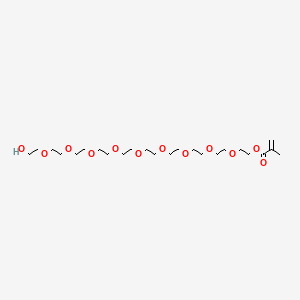
Poly(ethylene glycol)(N)monomethacrylate
Overview
Description
Poly(ethylene glycol)(N)monomethacrylate, also known as PEGMA, is a long-chain hydrophilic macromonomer . It is used to introduce hydrophilic sites into polymers, stabilize emulsion polymers, and prepare comb polymers . It is a biocompatible homopolymer with a brush type structure that is mainly used to provide a PEG modified surface .
Synthesis Analysis
PEGMA is synthesized by polymerization of ethylene oxide molecules to make joining units of ethylene glycol by an ether linkage . It is used to introduce hydrophilic sites into polymers, stabilize polymer emulsions, and in the synthesis of comb polymers .Molecular Structure Analysis
The linear formula of PEGMA is H2C=C(CH3)CO(OCH2CH2)nOH . The molecular weight of the PEG block is 400 .Chemical Reactions Analysis
PEGMA is used in various chemical reactions. For instance, it is used in the immobilization and release of the redox mediator ferrocene monocarboxylic acid from within cross-linked p(HEMA-co-PEGMA-co-HMMA) hydrogels . It is also used in a novel approach for the control of drug release rate through hydrogel membrane .Physical And Chemical Properties Analysis
PEGMA has a refractive index of n20/D 1.467 and a density of 1.101 g/mL at 25°C . The molecular weight of the PEG block is 400 .Scientific Research Applications
Biomedical Applications
Poly(ethylene glycol) methacrylate (PEGMA) hydrolyzable microspheres have been developed for biomedical applications, specifically for transient vascular embolization. These microspheres, formed using a suspension polymerization process, exhibit rapid hydrolytic degradation, producing low-molecular-weight compounds and water-soluble polymethacrylate chains. They have been shown to exhibit minimal cytotoxicity against L929 fibroblasts and to provoke only a mild and transient inflammatory reaction in vivo in a rabbit model (Louguet et al., 2014).
Pharmaceutical Fields
Poly(methacrylic acid-g-ethylene glycol) (P(MAA-g-EG)), which is pH-responsive, has shown promise in the biomedical and pharmaceutical fields, particularly as an oral carrier for peptide and protein drugs (Robinson & Peppas, 2002).
PEGylation Chemistry
PEGylation, the covalent attachment of PEG to peptides and proteins, is significant in pharmaceutical applications. It enhances the properties of peptides and proteins by shielding antigenic and immunogenic epitopes, preventing degradation by proteolytic enzymes, and altering biodistribution. This process involves incorporating various PEG functional groups to attach PEG to peptides or proteins (Roberts et al., 2002).
Mechanism of Action
Target of Action
PEG methacrylate, also known as Methacrylate-PEG-Hydroxy or Poly(ethylene glycol)(N)monomethacrylate, is a versatile building block in the field of polymer chemistry . It doesn’t have a specific biological target, but it’s primarily used to modify the properties of various substances, including drugs and other polymers . The primary targets of PEG methacrylate are therefore the substances it is combined with, altering their properties to enhance their functionality .
Mode of Action
The mode of action of PEG methacrylate involves its reaction with other substances to form new compounds with desired properties . For instance, PEG methacrylate can react with nucleophilic agents to yield new linear homo/co-polymers . The materials obtained have different physicochemical properties depending on the nucleophiles utilized . This allows for the creation of a wide variety of chemically diverse materials characterized by controlled structure and specific functions .
Biochemical Pathways
PEG methacrylate doesn’t directly interact with biochemical pathways. Instead, it modifies the properties of substances that do . For example, when used in the creation of drug nanocarriers, PEG methacrylate can enhance the pharmacokinetics of the drugs . This can indirectly affect the biochemical pathways that these drugs interact with by improving their delivery and effectiveness .
Pharmacokinetics
The pharmacokinetics of PEG methacrylate are largely dependent on the substances it is combined with . When used in drug delivery systems, PEG methacrylate can improve the Absorption, Distribution, Metabolism, and Excretion (ADME) properties of the drugs . This can enhance their bioavailability and therapeutic effectiveness .
Result of Action
The result of PEG methacrylate’s action is the creation of new compounds with enhanced properties . For example, when used in the creation of hydrogels, PEG methacrylate can improve their mechanical response and biocompatibility . This makes them suitable for various applications in the biomedical field, such as tissue engineering and drug delivery .
Action Environment
The action environment of PEG methacrylate can influence its reactivity and the properties of the compounds it forms . For instance, the presence of certain environmental factors, such as temperature and pH, can affect the polymerization process of PEG methacrylate . This can in turn influence the properties of the resulting polymers and their effectiveness in their intended applications .
Safety and Hazards
Future Directions
While specific future directions for PEGMA are not mentioned in the search results, its use in various fields such as medical and industrial areas, and its role in the synthesis of comb polymers and stabilization of emulsion polymers, suggest that it will continue to be a significant material in polymer chemistry .
Relevant Papers
- "Immobilization and release of the redox mediator ferrocene monocarboxylic acid from within cross-linked p(HEMA-co-PEGMA-co-HMMA) hydrogels" .
- "A novel approach for the control of drug release rate through hydrogel membrane: I. Effect of drug immobilization on drug release rate by copolymerization method" .
properties
IUPAC Name |
2-[2-[2-[2-[2-[2-[2-[2-[2-(2-hydroxyethoxy)ethoxy]ethoxy]ethoxy]ethoxy]ethoxy]ethoxy]ethoxy]ethoxy]ethyl 2-methylprop-2-enoate | |
---|---|---|
Details | Computed by Lexichem TK 2.7.0 (PubChem release 2021.05.07) | |
Source | PubChem | |
URL | https://pubchem.ncbi.nlm.nih.gov | |
Description | Data deposited in or computed by PubChem | |
InChI |
InChI=1S/C24H46O12/c1-23(2)24(26)36-22-21-35-20-19-34-18-17-33-16-15-32-14-13-31-12-11-30-10-9-29-8-7-28-6-5-27-4-3-25/h25H,1,3-22H2,2H3 | |
Details | Computed by InChI 1.0.6 (PubChem release 2021.05.07) | |
Source | PubChem | |
URL | https://pubchem.ncbi.nlm.nih.gov | |
Description | Data deposited in or computed by PubChem | |
InChI Key |
ANVPMFOXHJVWBT-UHFFFAOYSA-N | |
Details | Computed by InChI 1.0.6 (PubChem release 2021.05.07) | |
Source | PubChem | |
URL | https://pubchem.ncbi.nlm.nih.gov | |
Description | Data deposited in or computed by PubChem | |
Canonical SMILES |
CC(=C)C(=O)OCCOCCOCCOCCOCCOCCOCCOCCOCCOCCO | |
Details | Computed by OEChem 2.3.0 (PubChem release 2021.05.07) | |
Source | PubChem | |
URL | https://pubchem.ncbi.nlm.nih.gov | |
Description | Data deposited in or computed by PubChem | |
Molecular Formula |
C24H46O12 | |
Details | Computed by PubChem 2.1 (PubChem release 2021.05.07) | |
Source | PubChem | |
URL | https://pubchem.ncbi.nlm.nih.gov | |
Description | Data deposited in or computed by PubChem | |
Molecular Weight |
526.6 g/mol | |
Details | Computed by PubChem 2.1 (PubChem release 2021.05.07) | |
Source | PubChem | |
URL | https://pubchem.ncbi.nlm.nih.gov | |
Description | Data deposited in or computed by PubChem | |
Physical Description |
Liquid; [Sigma-Aldrich MSDS] | |
Record name | Poly(ethylene glycol) methacrylate | |
Source | Haz-Map, Information on Hazardous Chemicals and Occupational Diseases | |
URL | https://haz-map.com/Agents/21520 | |
Description | Haz-Map® is an occupational health database designed for health and safety professionals and for consumers seeking information about the adverse effects of workplace exposures to chemical and biological agents. | |
Explanation | Copyright (c) 2022 Haz-Map(R). All rights reserved. Unless otherwise indicated, all materials from Haz-Map are copyrighted by Haz-Map(R). No part of these materials, either text or image may be used for any purpose other than for personal use. Therefore, reproduction, modification, storage in a retrieval system or retransmission, in any form or by any means, electronic, mechanical or otherwise, for reasons other than personal use, is strictly prohibited without prior written permission. | |
CAS RN |
25736-86-1 | |
Record name | Poly(oxy-1,2-ethanediyl), .alpha.-(2-methyl-1-oxo-2-propen-1-yl)-.omega.-hydroxy- | |
Source | EPA Chemicals under the TSCA | |
URL | https://www.epa.gov/chemicals-under-tsca | |
Description | EPA Chemicals under the Toxic Substances Control Act (TSCA) collection contains information on chemicals and their regulations under TSCA, including non-confidential content from the TSCA Chemical Substance Inventory and Chemical Data Reporting. | |
Record name | 2-Hydroxyethyl methacrylate, ethoxylated | |
Source | European Chemicals Agency (ECHA) | |
URL | https://echa.europa.eu/substance-information/-/substanceinfo/100.105.550 | |
Description | The European Chemicals Agency (ECHA) is an agency of the European Union which is the driving force among regulatory authorities in implementing the EU's groundbreaking chemicals legislation for the benefit of human health and the environment as well as for innovation and competitiveness. | |
Explanation | Use of the information, documents and data from the ECHA website is subject to the terms and conditions of this Legal Notice, and subject to other binding limitations provided for under applicable law, the information, documents and data made available on the ECHA website may be reproduced, distributed and/or used, totally or in part, for non-commercial purposes provided that ECHA is acknowledged as the source: "Source: European Chemicals Agency, http://echa.europa.eu/". Such acknowledgement must be included in each copy of the material. ECHA permits and encourages organisations and individuals to create links to the ECHA website under the following cumulative conditions: Links can only be made to webpages that provide a link to the Legal Notice page. | |
Retrosynthesis Analysis
AI-Powered Synthesis Planning: Our tool employs the Template_relevance Pistachio, Template_relevance Bkms_metabolic, Template_relevance Pistachio_ringbreaker, Template_relevance Reaxys, Template_relevance Reaxys_biocatalysis model, leveraging a vast database of chemical reactions to predict feasible synthetic routes.
One-Step Synthesis Focus: Specifically designed for one-step synthesis, it provides concise and direct routes for your target compounds, streamlining the synthesis process.
Accurate Predictions: Utilizing the extensive PISTACHIO, BKMS_METABOLIC, PISTACHIO_RINGBREAKER, REAXYS, REAXYS_BIOCATALYSIS database, our tool offers high-accuracy predictions, reflecting the latest in chemical research and data.
Strategy Settings
Precursor scoring | Relevance Heuristic |
---|---|
Min. plausibility | 0.01 |
Model | Template_relevance |
Template Set | Pistachio/Bkms_metabolic/Pistachio_ringbreaker/Reaxys/Reaxys_biocatalysis |
Top-N result to add to graph | 6 |
Feasible Synthetic Routes
Disclaimer and Information on In-Vitro Research Products
Please be aware that all articles and product information presented on BenchChem are intended solely for informational purposes. The products available for purchase on BenchChem are specifically designed for in-vitro studies, which are conducted outside of living organisms. In-vitro studies, derived from the Latin term "in glass," involve experiments performed in controlled laboratory settings using cells or tissues. It is important to note that these products are not categorized as medicines or drugs, and they have not received approval from the FDA for the prevention, treatment, or cure of any medical condition, ailment, or disease. We must emphasize that any form of bodily introduction of these products into humans or animals is strictly prohibited by law. It is essential to adhere to these guidelines to ensure compliance with legal and ethical standards in research and experimentation.