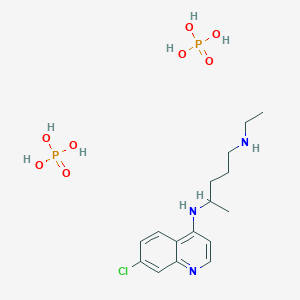
Desethyl chloroquine diphosphate
Overview
Description
Desethyl chloroquine diphosphate (DCQDP) is a primary metabolite of chloroquine diphosphate (CQDP), a 4-aminoquinoline antimalarial drug. Structurally, DCQDP lacks one ethyl group compared to CQDP (C₁₆H₂₂ClN₃ vs. C₁₈H₂₆ClN₃ for CQDP) . Its molecular weight is 291.81898 g/mol, and it exists as a solid at room temperature with a density of 1.138 g/cm³ . DCQDP is pharmacologically active, contributing to the prolonged efficacy of chloroquine therapy due to its long terminal half-life (>42 days in dogs) . It is detectable in blood alongside chloroquine and bisdesethylchloroquine (BDCQ), a secondary metabolite, for weeks post-administration .
Preparation Methods
Synthetic Routes and Reaction Conditions
Desethyl chloroquine (diphosphate) is synthesized through the desethylation of chloroquine. The process involves the removal of an ethyl group from chloroquine, typically using chemical reagents under controlled conditions. The reaction conditions often include the use of strong acids or bases to facilitate the desethylation process .
Industrial Production Methods
Industrial production of desethyl chloroquine (diphosphate) involves large-scale chemical synthesis using optimized reaction conditions to ensure high yield and purity. The process may include steps such as purification through crystallization or chromatography to isolate the desired compound .
Chemical Reactions Analysis
Types of Reactions
Desethyl chloroquine (diphosphate) undergoes various chemical reactions, including:
Oxidation: The compound can be oxidized to form different metabolites.
Reduction: Reduction reactions can modify the chemical structure, potentially altering its pharmacological properties.
Substitution: Substitution reactions can introduce new functional groups into the molecule, affecting its activity.
Common Reagents and Conditions
Common reagents used in these reactions include oxidizing agents like hydrogen peroxide, reducing agents such as sodium borohydride, and various catalysts to facilitate substitution reactions. The conditions often involve controlled temperatures and pH levels to ensure the desired reaction outcome .
Major Products
The major products formed from these reactions include various metabolites and derivatives of desethyl chloroquine (diphosphate), which may have different pharmacological activities and properties .
Scientific Research Applications
Pharmacological Properties
Desethyl chloroquine diphosphate exhibits several pharmacological activities that contribute to its therapeutic potential:
- Antimalarial Activity : As a metabolite of chloroquine, DCQ-DP retains antimalarial properties by interfering with the heme detoxification process in Plasmodium species. It inhibits the formation of hemozoin, leading to toxic heme accumulation that is lethal to the parasite .
- Inhibition of Autophagy : DCQ-DP is recognized as an inhibitor of autophagy, a cellular degradation process that can be exploited by some pathogens to survive within host cells. This property may enhance its effectiveness against certain infections .
- Modulation of Toll-like Receptors (TLRs) : The compound has been shown to interact with TLRs, which play a critical role in the immune response. By modulating these receptors, DCQ-DP may influence inflammatory pathways and immune responses .
Clinical Applications
This compound has been explored in various clinical contexts:
- Malaria Treatment : Similar to chloroquine, DCQ-DP is effective against malaria-causing parasites. Its use is particularly relevant in regions where resistance to traditional antimalarials has developed. Studies indicate that DCQ-DP may be effective against resistant strains of Plasmodium falciparum .
- Autoimmune Disorders : Chloroquine and its metabolites, including DCQ-DP, have been used in managing autoimmune diseases such as rheumatoid arthritis and systemic lupus erythematosus (SLE). They are believed to exert immunomodulatory effects that alleviate symptoms associated with these conditions .
Malaria Resistance
A study highlighted the effectiveness of this compound against chloroquine-resistant strains of Plasmodium falciparum. Researchers found that DCQ-DP maintained activity where chloroquine failed, suggesting it could be a viable alternative in treatment protocols .
Autoimmune Conditions
Clinical trials have demonstrated that patients with rheumatoid arthritis treated with chloroquine derivatives showed significant improvement in disease symptoms. The role of DCQ-DP as an active metabolite was emphasized, indicating its contribution to the therapeutic effects observed in these patients .
Antiviral Properties
Research has also indicated potential antiviral effects of this compound. By raising the pH within endosomes, it may inhibit the entry and replication of certain viruses, making it a candidate for further investigation in viral infections .
Data Summary
Application Area | Mechanism of Action | Clinical Evidence |
---|---|---|
Antimalarial | Inhibits heme detoxification | Effective against resistant Plasmodium strains |
Autoimmune Disorders | Immunomodulation | Improves symptoms in RA and SLE patients |
Viral Infections | Raises endosomal pH inhibiting viral entry | Potential antiviral activity noted |
Mechanism of Action
Desethyl chloroquine (diphosphate) exerts its effects by inhibiting the action of heme polymerase in malarial trophozoites, preventing the conversion of heme to hemazoin. This leads to the accumulation of toxic heme, which ultimately kills the parasite . Additionally, the compound may affect other molecular targets and pathways, contributing to its pharmacological activity .
Comparison with Similar Compounds
Comparison with Chloroquine Diphosphate
Structural and Physicochemical Differences
Property | Chloroquine Diphosphate (CQDP) | Desethyl Chloroquine Diphosphate (DCQDP) |
---|---|---|
Molecular Formula | C₁₈H₂₆ClN₃·2H₃PO₄ | C₁₆H₂₂ClN₃·2H₃PO₄ |
Molecular Weight | 515.86 g/mol | 291.82 g/mol (free base) |
CAS Number | 50-63-5 | 247912-76-1 |
Appearance | White crystalline powder | Solid (specific form not detailed) |
Solubility | Highly soluble in water | Limited data; inferred lower solubility |
CQDP’s solubility in organic solvents (e.g., ethanol, methanol) has been extensively studied , while DCQDP’s solubility data remain sparse.
Pharmacokinetics
- Half-Life : CQDP exhibits a terminal half-life of 12.6–14.5 days in dogs, while DCQDP persists in blood for up to 42 days, suggesting a similarly prolonged half-life .
- Volume of Distribution (Vdss) : CQDP has a Vdss of 53.3 L/kg, indicating extensive tissue distribution . DCQDP’s Vdss is unreported but likely comparable due to structural similarity.
- Clearance : CQDP’s blood clearance is 2.67 L/kg/day . DCQDP clearance data are unavailable.
Antimalarial Activity
Strain Type | CQDP Activity (IC₅₀) | DCQDP Activity (IC₅₀) |
---|---|---|
Chloroquine-sensitive P. falciparum | High | Equipotent to CQDP |
Chloroquine-resistant P. falciparum | Reduced | Less active than CQDP |
DCQDP retains full activity against chloroquine-sensitive strains but shows reduced efficacy against resistant strains compared to CQDP . This suggests that the ethyl group in CQDP may enhance binding to resistant parasite targets.
Comparison with Bisdesethylchloroquine (BDCQ)
BDCQ, a secondary metabolite lacking both ethyl groups of CQDP, exhibits significantly lower antimalarial activity:
- Chloroquine-sensitive strains : BDCQ is less active than both CQDP and DCQDP.
The progressive loss of ethyl groups (CQDP → DCQDP → BDCQ) correlates with diminished efficacy, highlighting the importance of alkyl side chains in 4-aminoquinoline antimalarials.
Analytical Methods for Detection
A validated HPLC-MS method simultaneously quantifies CQDP and DCQDP in human plasma, with linear ranges of 2.03–502.57 ng/mL for CQDP and 2.02–501.73 ng/mL for DCQDP . This underscores their coexistence in biological systems and the need for precise monitoring during therapy.
Biological Activity
Desethyl chloroquine diphosphate (DCQ) is a significant metabolite of chloroquine, an antimalarial drug that has been extensively studied for its biological activities. This article delves into the biological activity of DCQ, focusing on its pharmacokinetics, mechanisms of action, and therapeutic implications.
Overview of this compound
Desethyl chloroquine is primarily formed through the N-dealkylation of chloroquine by cytochrome P450 enzymes, particularly CYP2C8 and CYP3A4 . It retains some biological activities similar to its parent compound but exhibits distinct pharmacological profiles that warrant further exploration.
Pharmacokinetics
The pharmacokinetics of DCQ are crucial for understanding its efficacy and safety. Key parameters include:
- Bioavailability : DCQ has a variable bioavailability influenced by the formulation and patient factors. In studies, the bioavailability of chloroquine (and consequently DCQ) ranged from 52% to 114% when administered orally .
- Plasma Concentrations : In clinical trials, median plasma concentrations of DCQ were observed to be approximately 206 ng/mL after 10 weeks of treatment with chloroquine . The ratio of chloroquine to desethyl chloroquine was also noted to vary significantly among different treatment regimens.
Time Point | Chloroquine (ng/mL) | Desethyl Chloroquine (ng/mL) | Ratio (CQ:DCQ) |
---|---|---|---|
Week 10 | 214 (139–279) | 206 (94–332) | 1.04 |
Week 12 | 210 (159–290) | 208 (135–406) | 1.05 |
DCQ functions through several mechanisms that contribute to its biological activity:
- Antimalarial Activity : Like chloroquine, DCQ inhibits heme polymerase in Plasmodium species, leading to the accumulation of toxic heme within the parasite . This mechanism is critical for its effectiveness against malaria.
- Inhibition of Viral Entry : Recent studies indicate that DCQ can inhibit the glycosylation of ACE2, a receptor utilized by viruses like SARS-CoV-2 for cell entry. This inhibition may reduce viral load and improve outcomes in viral infections .
- Immunomodulatory Effects : DCQ has been shown to modulate immune responses by inhibiting various pro-inflammatory cytokines and chemokines, which could have implications for treating autoimmune diseases .
Case Studies and Clinical Findings
Several studies have highlighted the clinical relevance of DCQ:
- Antiretroviral Therapy Interaction : A study involving patients on antiretroviral therapy showed altered plasma concentrations of both chloroquine and DCQ, suggesting potential interactions that could affect therapeutic outcomes .
- Distribution in Blood Cells : Research indicated that about 70-85% of chloroquine and its metabolites, including DCQ, are concentrated in blood cells rather than plasma. This finding suggests a significant role for blood cell binding in the drug's pharmacodynamics .
- Efficacy Against Resistant Strains : New analogs of chloroquine that include modifications targeting DCQ have shown promising results against chloroquine-resistant strains of Plasmodium falciparum, indicating potential avenues for developing more effective antimalarial therapies .
Q & A
Basic Research Questions
Q. How is desethyl chloroquine diphosphate structurally identified and quantified in biological matrices?
this compound, a primary metabolite of chloroquine, can be quantified using reversed-phase high-performance liquid chromatography (HPLC) with ultraviolet (UV) detection. A validated method involves a one-step extraction using chlorpheniramine as an internal standard, enabling simultaneous detection in plasma, whole blood, and urine . The retention time and UV spectral properties (e.g., λmax ~254 nm) differentiate it from chloroquine and other metabolites. Calibration curves must account for matrix effects (e.g., plasma proteins) to ensure accuracy .
Q. What are the primary biological activities of this compound in preclinical studies?
this compound retains antimalarial activity by inhibiting heme polymerization in Plasmodium species, though with reduced potency compared to its parent compound, chloroquine . It also acts as an autophagy inhibitor by raising lysosomal pH, blocking autophagosome-lysosome fusion, which is leveraged in cancer research to study autophagy-dependent pathways .
Q. What analytical techniques are recommended for distinguishing this compound from related metabolites?
Liquid chromatography-tandem mass spectrometry (LC-MS/MS) with multiple reaction monitoring (MRM) provides high specificity. Key transitions include m/z 292.1 → 245.1 (desethyl chloroquine) and m/z 516.1 → 291.8 (chloroquine diphosphate). Chromatographic separation on a C18 column with ion-pairing agents (e.g., trifluoroacetic acid) resolves co-eluting metabolites .
Advanced Research Questions
Q. How does this compound modulate autophagy flux in in vivo models, and what are the critical experimental parameters?
In transgenic Huntington’s disease (HD) mice, this compound (10 mg/kg/day, intraperitoneal) reduces autophagy flux by accumulating LC3-II and p62 in brain tissue. Researchers must standardize dosing schedules, tissue collection times, and use Western blotting with antibodies specific to autophagy markers (e.g., anti-LC3B). Control groups should include chloroquine-treated animals to compare metabolite-specific effects .
Q. How should pharmacokinetic studies be designed to account for this compound’s variable tissue distribution?
Key steps include:
- Sampling: Collect plasma, erythrocytes, and tissues (liver, spleen) at multiple time points (0–72 hrs post-dose) to capture distribution kinetics.
- Analytical Validation: Use deuterated internal standards (e.g., desethyl chloroquine-d4) to correct for ion suppression in mass spectrometry .
- Modeling: Apply compartmental pharmacokinetic models to estimate clearance (CL) and volume of distribution (Vd), noting species-specific differences in metabolic enzymes (e.g., CYP2D6 activity) .
Q. How can researchers resolve discrepancies in reported IC50 values for this compound’s cytotoxicity?
Variability in cytotoxicity assays (e.g., MTT, ATP luminescence) often stems from differences in:
- Cell Lines: Human KB cells show higher sensitivity (IC50 ~43.9 μM) than murine macrophages (IC50 >140 μM) due to differential lysosomal uptake .
- Assay Duration: Prolonged exposure (48–72 hrs) increases potency by enhancing lysosomal accumulation.
- Standardization: Normalize data to cell count (via DNA quantification) rather than optical density to mitigate interference from metabolite precipitation .
Q. What strategies mitigate off-target effects when studying this compound’s role in TLR inhibition?
To isolate Toll-like receptor (TLR) inhibition from autophagy effects:
- Use TLR-specific knockout cell lines (e.g., HEK293-TLR4−/−) alongside wild-type controls.
- Pair this compound with selective autophagy inducers (e.g., rapamycin) to decouple TLR signaling from autophagy modulation .
Q. How can researchers validate this compound’s stability under different storage conditions?
Stability studies should assess:
- Temperature: Store lyophilized powder at −80°C for long-term stability; avoid freeze-thaw cycles for reconstituted solutions.
- pH Sensitivity: Use phosphate-buffered saline (PBS, pH 7.4) instead of unbuffered solutions to prevent degradation.
- Analytical Confirmation: Monitor degradation products (e.g., didesethyl chloroquine) via LC-MS/MS over 24–48 hrs .
Q. Methodological Considerations
- Sample Preparation: For tissue homogenates, use protein precipitation with acetonitrile (3:1 v/v) followed by centrifugation (14,000 ×g, 10 min) to minimize matrix interference .
- Ethical Reporting: Disclose metabolite purity (≥98% by HPLC) and supplier lot numbers to ensure reproducibility, avoiding non-validated sources (e.g., ) .
Properties
IUPAC Name |
4-N-(7-chloroquinolin-4-yl)-1-N-ethylpentane-1,4-diamine;phosphoric acid | |
---|---|---|
Details | Computed by LexiChem 2.6.6 (PubChem release 2019.06.18) | |
Source | PubChem | |
URL | https://pubchem.ncbi.nlm.nih.gov | |
Description | Data deposited in or computed by PubChem | |
InChI |
InChI=1S/C16H22ClN3.2H3O4P/c1-3-18-9-4-5-12(2)20-15-8-10-19-16-11-13(17)6-7-14(15)16;2*1-5(2,3)4/h6-8,10-12,18H,3-5,9H2,1-2H3,(H,19,20);2*(H3,1,2,3,4) | |
Details | Computed by InChI 1.0.5 (PubChem release 2019.06.18) | |
Source | PubChem | |
URL | https://pubchem.ncbi.nlm.nih.gov | |
Description | Data deposited in or computed by PubChem | |
InChI Key |
PIKNKEKUPZTKFZ-UHFFFAOYSA-N | |
Details | Computed by InChI 1.0.5 (PubChem release 2019.06.18) | |
Source | PubChem | |
URL | https://pubchem.ncbi.nlm.nih.gov | |
Description | Data deposited in or computed by PubChem | |
Canonical SMILES |
CCNCCCC(C)NC1=C2C=CC(=CC2=NC=C1)Cl.OP(=O)(O)O.OP(=O)(O)O | |
Details | Computed by OEChem 2.1.5 (PubChem release 2019.06.18) | |
Source | PubChem | |
URL | https://pubchem.ncbi.nlm.nih.gov | |
Description | Data deposited in or computed by PubChem | |
Molecular Formula |
C16H28ClN3O8P2 | |
Details | Computed by PubChem 2.1 (PubChem release 2019.06.18) | |
Source | PubChem | |
URL | https://pubchem.ncbi.nlm.nih.gov | |
Description | Data deposited in or computed by PubChem | |
DSSTOX Substance ID |
DTXSID70678694 | |
Record name | Phosphoric acid--N~4~-(7-chloroquinolin-4-yl)-N~1~-ethylpentane-1,4-diamine (2/1) | |
Source | EPA DSSTox | |
URL | https://comptox.epa.gov/dashboard/DTXSID70678694 | |
Description | DSSTox provides a high quality public chemistry resource for supporting improved predictive toxicology. | |
Molecular Weight |
487.8 g/mol | |
Details | Computed by PubChem 2.1 (PubChem release 2021.05.07) | |
Source | PubChem | |
URL | https://pubchem.ncbi.nlm.nih.gov | |
Description | Data deposited in or computed by PubChem | |
CAS No. |
247912-76-1 | |
Record name | Phosphoric acid--N~4~-(7-chloroquinolin-4-yl)-N~1~-ethylpentane-1,4-diamine (2/1) | |
Source | EPA DSSTox | |
URL | https://comptox.epa.gov/dashboard/DTXSID70678694 | |
Description | DSSTox provides a high quality public chemistry resource for supporting improved predictive toxicology. | |
Retrosynthesis Analysis
AI-Powered Synthesis Planning: Our tool employs the Template_relevance Pistachio, Template_relevance Bkms_metabolic, Template_relevance Pistachio_ringbreaker, Template_relevance Reaxys, Template_relevance Reaxys_biocatalysis model, leveraging a vast database of chemical reactions to predict feasible synthetic routes.
One-Step Synthesis Focus: Specifically designed for one-step synthesis, it provides concise and direct routes for your target compounds, streamlining the synthesis process.
Accurate Predictions: Utilizing the extensive PISTACHIO, BKMS_METABOLIC, PISTACHIO_RINGBREAKER, REAXYS, REAXYS_BIOCATALYSIS database, our tool offers high-accuracy predictions, reflecting the latest in chemical research and data.
Strategy Settings
Precursor scoring | Relevance Heuristic |
---|---|
Min. plausibility | 0.01 |
Model | Template_relevance |
Template Set | Pistachio/Bkms_metabolic/Pistachio_ringbreaker/Reaxys/Reaxys_biocatalysis |
Top-N result to add to graph | 6 |
Feasible Synthetic Routes
Disclaimer and Information on In-Vitro Research Products
Please be aware that all articles and product information presented on BenchChem are intended solely for informational purposes. The products available for purchase on BenchChem are specifically designed for in-vitro studies, which are conducted outside of living organisms. In-vitro studies, derived from the Latin term "in glass," involve experiments performed in controlled laboratory settings using cells or tissues. It is important to note that these products are not categorized as medicines or drugs, and they have not received approval from the FDA for the prevention, treatment, or cure of any medical condition, ailment, or disease. We must emphasize that any form of bodily introduction of these products into humans or animals is strictly prohibited by law. It is essential to adhere to these guidelines to ensure compliance with legal and ethical standards in research and experimentation.