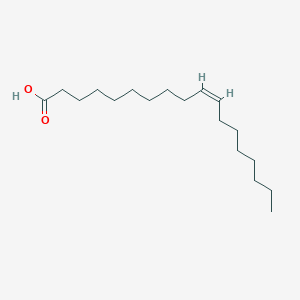
cis-10-Octadecenoic acid
Overview
Description
cis-10-Octadecenoic acid (CAS: 2442-70-8) is a monounsaturated fatty acid with the molecular formula C₁₈H₃₄O₂. It features a cis-configured double bond at the 10th carbon of its 18-carbon chain . Evidence suggests it interacts with core targets such as AKT1, MAPK3, and PPARG, implicating it in pathways like neurodegeneration and lipid metabolism .
Preparation Methods
Synthetic Routes and Reaction Conditions:
Hydrolysis and Methylation: Fatty acids, including cis-10-Octadecenoic acid, can be synthesized through hydrolysis of triglycerides followed by methylation to form fatty acid methyl esters (FAMEs).
Biological Synthesis: This compound can also be produced biologically through the hydration of linoleic acid by specific enzymes such as linoleate hydratase found in certain lactic acid bacteria.
Industrial Production Methods:
Extraction from Natural Sources: this compound can be extracted from natural sources such as plant oils using solvent extraction methods followed by purification processes like distillation and chromatography.
Chemical Synthesis: Industrial production may involve chemical synthesis routes that include the selective hydrogenation of polyunsaturated fatty acids to yield the desired monounsaturated fatty acid.
Chemical Reactions Analysis
Types of Reactions:
Oxidation: cis-10-Octadecenoic acid can undergo oxidation reactions to form hydroxy and oxo fatty acids.
Hydration: The compound can be hydrated to form hydroxy fatty acids under the action of specific enzymes.
Hydrogenation: It can be hydrogenated to form stearic acid, a saturated fatty acid.
Common Reagents and Conditions:
Oxidation: Common oxidizing agents include potassium permanganate (KMnO4) and osmium tetroxide (OsO4).
Hydration: Enzymatic hydration typically involves linoleate hydratase.
Hydrogenation: Catalysts such as palladium on carbon (Pd/C) are used for hydrogenation reactions.
Major Products Formed:
- 10-Hydroxy-cis-12-octadecenoic acid
- 10-Oxo-cis-12-octadecenoic acid
- Stearic acid
Scientific Research Applications
Nutritional Science
Fatty Acid Metabolism and Health Implications
Cis-10-octadecenoic acid plays a significant role in human metabolism. Research indicates that it is absorbed efficiently in the human body, with studies showing that both cis-10 and trans-10 isomers are equally well absorbed, but they exhibit different metabolic pathways. For instance, the cis isomer is oxidized more rapidly than its trans counterpart, which can influence lipid profiles in the body .
A notable study analyzed the uptake and turnover rates of deuterium-labeled this compound in human plasma. The findings revealed that these fatty acids were preferentially incorporated into specific positions within phosphatidylcholine, suggesting their potential role in membrane fluidity and function .
Table 1: Metabolic Characteristics of this compound
Characteristic | This compound | trans-10-Octadecenoic Acid |
---|---|---|
Absorption Efficiency | High | High |
Oxidation Rate | Rapid | Slower |
Incorporation into Phosphatidylcholine | Preferentially at 1-acyl position | Excluded from 2-acyl position |
Esterification with Cholesterol | Slower | Faster |
Pharmaceutical Applications
Potential Therapeutic Uses
Recent research has highlighted the therapeutic potential of this compound derivatives in treating metabolic disorders such as type II diabetes and non-alcoholic steatohepatitis (NASH). One promising derivative, 10-hydroxy-cis-12-octadecenoic acid (HYA) , produced from linoleic acid by gut microbiota, has been shown to improve insulin sensitivity through G protein-coupled receptors (GPCRs) expressed in the intestine .
Ongoing studies aim to develop pharmaceuticals based on HYA to mitigate chronic illnesses associated with obesity and metabolic syndrome. For example, a collaborative effort at Kyoto University is focusing on elucidating the metabolic pathways of HYA to enhance its clinical applications .
Industrial Applications
Food Industry
In the food industry, this compound is valuable for modifying fatty acid profiles in edible oils. The enzymatic conversion processes involving this fatty acid can lead to the production of healthier oils with reduced trans-fat content. Research has demonstrated that enzymes such as CLA-HY can catalyze reactions that enhance the ratio of beneficial cis fatty acids while minimizing harmful trans isomers .
Table 2: Enzymatic Reactions Involving this compound
Enzyme | Reaction Type | Product(s) Generated |
---|---|---|
CLA-HY | Isomerization | cis-9,trans-11-CLA; trans-9,trans-11-CLA |
CLA-DH | Hydroxylation | 10-hydroxy-cis-12-octadecenoic acid |
CLA-DC | Dehydration | Enhanced cis/trans ratio |
Case Studies
Case Study 1: Metabolic Effects in Humans
A study involving young male subjects assessed the metabolic effects of feeding them a mixture of triacylglycerols containing deuterated this compound. The results indicated significant differences in absorption and oxidation rates compared to other fatty acids, emphasizing the unique metabolic behavior of this compound .
Case Study 2: Pharmaceutical Development
Research led by Professor Ikuo Kimura at Kyoto University investigated the effects of HYA on insulin resistance in mouse models. The findings suggested that HYA could be a promising candidate for drug development aimed at treating type II diabetes, showcasing its potential beyond nutritional applications .
Mechanism of Action
cis-10-Octadecenoic acid exerts its effects primarily through its interaction with peroxisome proliferator-activated receptors (PPARs). These receptors regulate the expression of genes involved in fatty acid metabolism and energy homeostasis. Upon binding to PPARs, this compound can modulate the beta-oxidation pathway of fatty acids, influencing lipid metabolism and energy balance .
Comparison with Similar Compounds
Comparison with Structurally Similar Compounds
Structural and Chemical Properties
The table below compares cis-10-octadecenoic acid with other monounsaturated fatty acids (MUFAs) and derivatives:
Key Observations :
- Chain Length: cis-10-Heptadecenoic acid (C17) has a shorter chain than octadecenoic acids (C18), affecting melting points and solubility .
- Double Bond Position : Shifting the double bond from C9 (oleic acid) to C8 or C10 alters molecular packing and membrane fluidity. Enzymes like those from Pseudomonas sp. show specificity for cis-Δ9 bonds, making cis-10 less reactive in hydration/dehydration reactions .
Metabolic Pathways
- This compound: Implicated in Alzheimer’s disease via interactions with MAPK3 and PPARG, which regulate neuroinflammation and lipid homeostasis .
- Oleic acid (cis-9) : Well-characterized roles in energy storage, membrane synthesis, and anti-inflammatory signaling through PPARA and PTGS2 .
- cis-8-Octadecenoic acid: Shares targets with cis-10 (e.g., ABCB1) but shows distinct binding affinities due to double bond position .
Enzymatic Specificity
- The Pseudomonas enzyme catalyzes cis-Δ9 hydration and trans-Δ10 isomerization but cannot act directly on cis-10 .
- Primary kinetic isotope effects confirm that hydrogen addition/removal during isomerization is stereospecific, favoring cis-Δ9 substrates .
Biological Activity
Cis-10-octadecenoic acid, commonly known as cis-10-18:1 , is a monounsaturated fatty acid that has garnered attention for its various biological activities. This article reviews the compound's metabolic pathways, physiological effects, and potential therapeutic applications based on a range of scientific studies.
Chemical Structure and Properties
This compound is characterized by a double bond located at the 10th carbon of the octadecene chain. Its chemical formula is , and it is classified as an unsaturated fatty acid. The unique positioning of the double bond influences its biological behavior, particularly in lipid metabolism and cellular functions.
Metabolism and Bioavailability
Research indicates that this compound is metabolized in the human body similarly to other fatty acids. A study involving deuterium-labeled this compound showed that it was absorbed efficiently in the intestines, with turnover rates estimated between 3.47-5.13 mg/min per kg of body weight. The study highlighted that both cis-10 and trans-10 isomers were absorbed equally well, but the cis isomer exhibited faster oxidation rates compared to oleic acid (cis-9-octadecenoic acid) .
Biological Activities
1. Anti-inflammatory Effects
this compound has been implicated in anti-inflammatory pathways. A study demonstrated that metabolites derived from gut bacteria, including those from this compound, modulate immune responses by suppressing T cell proliferation and dendritic cell activation . This suggests potential therapeutic applications in inflammatory diseases.
2. Antimicrobial Properties
The compound has shown antimicrobial activity, particularly against certain pathogens. Research indicated that octadecenoic acids can inhibit enzyme activities in microbes, impair nutrient uptake, and induce oxidative stress leading to cell lysis . This property could be harnessed for developing natural antimicrobial agents.
3. Lipid Profile Modulation
this compound influences lipid profiles by preferentially incorporating into phosphatidylcholine at specific positions, affecting membrane fluidity and function . Its incorporation into triacylglycerols also alters cholesterol esterification rates, suggesting a role in cardiovascular health.
Case Studies and Research Findings
Q & A
Basic Research Questions
Q. What spectroscopic methods are most effective for characterizing the structure and purity of cis-10-Octadecenoic acid?
- Methodology : Nuclear Magnetic Resonance (NMR) spectroscopy is critical for confirming the position of the double bond (e.g., -NMR to identify proton shifts at C10) and stereochemistry. Gas Chromatography-Mass Spectrometry (GC-MS) quantifies purity and detects isomerization by comparing retention times with standards. Infrared (IR) spectroscopy can validate functional groups (e.g., carboxylic acid O-H stretch at ~2500–3300 cm). Cross-reference spectral libraries and use internal standards to minimize experimental bias .
Q. How does the double bond position in cis-10-Octadecenoic acid affect its physical properties compared to other octadecenoic acid isomers?
- Methodology : Compare melting points, solubility, and phase behavior via differential scanning calorimetry (DSC) and polarimetry. For example, cis-9-Octadecenoic acid (oleic acid) has a lower melting point (−4°C) due to reduced molecular symmetry, whereas cis-10 isomers may exhibit distinct crystallization patterns. Solubility in organic solvents (e.g., hexane, ethanol) can be tested gravimetrically under controlled temperatures .
Q. What protocols minimize isomerization during isolation of cis-10-Octadecenoic acid from natural sources?
- Methodology : Use low-temperature extraction (e.g., cold ethanol or acetone precipitation) to prevent thermal degradation. Employ inert atmospheres (N or Ar) during solvent evaporation. Validate purity via thin-layer chromatography (TLC) with iodine vapor staining or silver nitrate-impregnated plates to detect unsaturated isomers. Store samples at −80°C in amber vials to avoid photochemical reactions .
Advanced Research Questions
Q. How can enzymatic pathways for cis-10-Octadecenoic acid biosynthesis be experimentally elucidated?
- Methodology : Use isotope-labeled precursors (e.g., -acetate) in cell cultures or recombinant enzyme assays to track carbon flux. Knockout or CRISPR-Cas9 silencing of candidate desaturases (e.g., Δ10-desaturases) in model organisms (e.g., Saccharomyces cerevisiae) can confirm enzymatic specificity. Pair with lipidomics (LC-MS/MS) to quantify intermediate metabolites .
Q. What statistical frameworks are optimal for analyzing dose-response effects of cis-10-Octadecenoic acid in cell-based assays?
- Methodology : Apply nonlinear regression models (e.g., four-parameter logistic curve) to calculate EC values. Use ANOVA with post-hoc Tukey tests to compare treatment groups. Validate assumptions (normality, homoscedasticity) via Shapiro-Wilk and Levene’s tests. For high-throughput data, employ machine learning pipelines (e.g., random forests) to identify outliers .
Q. How can molecular dynamics (MD) simulations model cis-10-Octadecenoic acid’s behavior in lipid bilayers?
- Methodology : Build bilayer systems using tools like CHARMM-GUI. Parameterize the force field (e.g., Lipid14/17) for accurate representation of double-bond geometry. Run simulations in GROMACS or NAMD under physiological conditions (310 K, 1 atm). Analyze membrane fluidity via lateral diffusion coefficients and order parameters (S) from deuterium NMR proxies .
Q. What strategies resolve contradictions in reported biological activities of cis-10-Octadecenoic acid across studies?
- Methodology : Conduct meta-analyses to assess heterogeneity sources (e.g., cell lines, purity thresholds). Use funnel plots to detect publication bias. Replicate key experiments with standardized protocols (e.g., ISO 9001 guidelines) and orthogonal assays (e.g., fluorescence-based vs. colorimetric viability tests). Report negative results transparently .
Q. Methodological Considerations
- Experimental Design : Apply the FINER criteria (Feasible, Interesting, Novel, Ethical, Relevant) to hypothesis formulation. For example, a study on anti-inflammatory effects should define controls (e.g., oleic acid as an isomer control) and ethical animal use protocols .
- Data Reproducibility : Archive raw data (e.g., NMR spectra, chromatograms) in FAIR-compliant repositories. Use electronic lab notebooks (ELNs) for real-time documentation. Include positive/negative controls in each experimental batch to detect procedural drift .
Q. Tables
Property | cis-10-Octadecenoic Acid | cis-9-Octadecenoic Acid (Oleic Acid) |
---|---|---|
CAS Number | 2442-70-8 | 112-80-1 |
Molecular Formula | CHO | CHO |
Double Bond Position | C10 | C9 |
Melting Point | Literature-dependent* | −4°C |
Common Analytical Tools | GC-MS, -NMR | GC-MS, IR |
*Note: Experimental determination recommended due to limited literature on cis-10 isomer .
Properties
IUPAC Name |
(Z)-octadec-10-enoic acid | |
---|---|---|
Details | Computed by LexiChem 2.6.6 (PubChem release 2019.06.18) | |
Source | PubChem | |
URL | https://pubchem.ncbi.nlm.nih.gov | |
Description | Data deposited in or computed by PubChem | |
InChI |
InChI=1S/C18H34O2/c1-2-3-4-5-6-7-8-9-10-11-12-13-14-15-16-17-18(19)20/h8-9H,2-7,10-17H2,1H3,(H,19,20)/b9-8- | |
Details | Computed by InChI 1.0.5 (PubChem release 2019.06.18) | |
Source | PubChem | |
URL | https://pubchem.ncbi.nlm.nih.gov | |
Description | Data deposited in or computed by PubChem | |
InChI Key |
QXJSBBXBKPUZAA-HJWRWDBZSA-N | |
Details | Computed by InChI 1.0.5 (PubChem release 2019.06.18) | |
Source | PubChem | |
URL | https://pubchem.ncbi.nlm.nih.gov | |
Description | Data deposited in or computed by PubChem | |
Canonical SMILES |
CCCCCCCC=CCCCCCCCCC(=O)O | |
Details | Computed by OEChem 2.1.5 (PubChem release 2019.06.18) | |
Source | PubChem | |
URL | https://pubchem.ncbi.nlm.nih.gov | |
Description | Data deposited in or computed by PubChem | |
Isomeric SMILES |
CCCCCCC/C=C\CCCCCCCCC(=O)O | |
Details | Computed by OEChem 2.1.5 (PubChem release 2019.06.18) | |
Source | PubChem | |
URL | https://pubchem.ncbi.nlm.nih.gov | |
Description | Data deposited in or computed by PubChem | |
Molecular Formula |
C18H34O2 | |
Details | Computed by PubChem 2.1 (PubChem release 2019.06.18) | |
Source | PubChem | |
URL | https://pubchem.ncbi.nlm.nih.gov | |
Description | Data deposited in or computed by PubChem | |
Molecular Weight |
282.5 g/mol | |
Details | Computed by PubChem 2.1 (PubChem release 2021.05.07) | |
Source | PubChem | |
URL | https://pubchem.ncbi.nlm.nih.gov | |
Description | Data deposited in or computed by PubChem | |
Retrosynthesis Analysis
AI-Powered Synthesis Planning: Our tool employs the Template_relevance Pistachio, Template_relevance Bkms_metabolic, Template_relevance Pistachio_ringbreaker, Template_relevance Reaxys, Template_relevance Reaxys_biocatalysis model, leveraging a vast database of chemical reactions to predict feasible synthetic routes.
One-Step Synthesis Focus: Specifically designed for one-step synthesis, it provides concise and direct routes for your target compounds, streamlining the synthesis process.
Accurate Predictions: Utilizing the extensive PISTACHIO, BKMS_METABOLIC, PISTACHIO_RINGBREAKER, REAXYS, REAXYS_BIOCATALYSIS database, our tool offers high-accuracy predictions, reflecting the latest in chemical research and data.
Strategy Settings
Precursor scoring | Relevance Heuristic |
---|---|
Min. plausibility | 0.01 |
Model | Template_relevance |
Template Set | Pistachio/Bkms_metabolic/Pistachio_ringbreaker/Reaxys/Reaxys_biocatalysis |
Top-N result to add to graph | 6 |
Feasible Synthetic Routes
Disclaimer and Information on In-Vitro Research Products
Please be aware that all articles and product information presented on BenchChem are intended solely for informational purposes. The products available for purchase on BenchChem are specifically designed for in-vitro studies, which are conducted outside of living organisms. In-vitro studies, derived from the Latin term "in glass," involve experiments performed in controlled laboratory settings using cells or tissues. It is important to note that these products are not categorized as medicines or drugs, and they have not received approval from the FDA for the prevention, treatment, or cure of any medical condition, ailment, or disease. We must emphasize that any form of bodily introduction of these products into humans or animals is strictly prohibited by law. It is essential to adhere to these guidelines to ensure compliance with legal and ethical standards in research and experimentation.