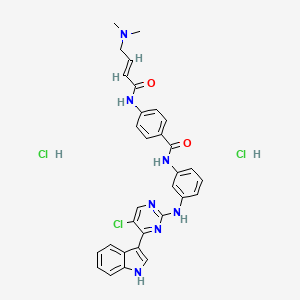
THZ1 Dihydrochloride
Overview
Description
THZ1 Dihydrochloride is a selective, covalent, and allosteric inhibitor of cyclin-dependent kinase 7 (CDK7). It has shown significant antiproliferative effects on various cancer cell lines. CDK7 plays a crucial role in regulating RNA polymerase-II-based transcription and promoting tumor progression .
Mechanism of Action
Target of Action
THZ1 Dihydrochloride, also known as THZ1 2HCl, is a potent and selective inhibitor of cyclin-dependent kinase 7 (CDK7) . CDK7 serves as a pivotal regulator in orchestrating cellular cycle dynamics and gene transcriptional activity . Elevated expression levels of CDK7 have been ubiquitously documented across a spectrum of malignancies and have been concomitantly correlated with adverse clinical outcomes . THZ1 achieves CDK7 specificity by targeting a remote cysteine residue located outside of the canonical kinase domain .
Mode of Action
THZ1 interacts with its primary target, CDK7, by combining allosteric covalent binding and ATP-site binding to irreversibly inhibit CDK7 . This inhibition leads to a pronounced downregulation of gene transcription, with a preferential repression of mitotic cell cycle and NF-κB signaling-related transcripts .
Biochemical Pathways
The inhibition of CDK7 by THZ1 affects several biochemical pathways. It specifically suppresses p38α/MYC-associated genes, leading to the downregulation of MYC transcriptional activity . This results in the suppression of the synthesis of two core transcription factors belonging to the hedgehog pathway (the glioma-associated oncogenes GLI1 and GLI2) .
Pharmacokinetics
While specific ADME (Absorption, Distribution, Metabolism, and Excretion) properties of this compound are not readily available, it’s known that THZ1 exhibits a potent inhibitory effect with an IC50 value of 3.2 nM . This suggests that the compound has a high binding affinity and could potentially have good bioavailability.
Result of Action
The inhibition of CDK7 by THZ1 leads to significant molecular and cellular effects. It elicits apoptosis and suppresses tumor growth . Moreover, THZ1 treatment results in the transcriptional modulation by inhibiting the phosphorylation of Ser2, Ser5, and Ser7 within RNA polymerase II (RNAPII) . This leads to the downregulation of several proteins, including MYC, MCL-1, and BCL-XL .
Action Environment
These can include lifestyle factors such as diet and exercise, as well as exposure to certain chemicals or pollutants . .
Biochemical Analysis
Biochemical Properties
THZ1 Dihydrochloride interacts with several enzymes and proteins. It is a potent inhibitor of CDK7, a kinase that plays a crucial role in cell cycle transitions and gene transcription . This compound achieves CDK7 specificity by targeting a remote cysteine residue located outside of the canonical kinase domain . This interaction leads to the inhibition of CDK7, thereby affecting the biochemical reactions that CDK7 is involved in .
Cellular Effects
This compound has significant effects on various types of cells and cellular processes. It has been shown to suppress tumor growth and elicit apoptosis in non-small cell lung cancer (NSCLC) cells . It also has a pronounced antineoplastic effect in gastrointestinal stromal tumor (GIST) cells . This compound influences cell function by inhibiting CDK7, which in turn suppresses the transcription of several genes, including those involved in cell signaling pathways, gene expression, and cellular metabolism .
Molecular Mechanism
The molecular mechanism of action of this compound involves its binding interactions with biomolecules and its effects on enzyme activity and gene expression. As a covalent inhibitor of CDK7, this compound binds to a remote cysteine residue on CDK7 . This binding inhibits the activity of CDK7, leading to the suppression of the transcription of several genes . This includes the downregulation of MYC, a gene that plays a crucial role in cell cycle progression, apoptosis, and cellular transformation .
Temporal Effects in Laboratory Settings
In laboratory settings, the effects of this compound change over time. It has been shown to have a dose-dependent inhibitory effect in various cancers . Over time, this compound can lead to cell cycle arrest, apoptosis induction, and DNA damage . It also suppresses the transcription of several genes over time, leading to long-term effects on cellular function .
Dosage Effects in Animal Models
The effects of this compound vary with different dosages in animal models. In a murine orthotopic pancreatic cancer model, the combined use of THZ1 and antiPD-1 therapy resulted in significant tumor regression
Metabolic Pathways
This compound is involved in several metabolic pathways. It inhibits CDK7, which plays a crucial role in the regulation of cell cycle transitions and gene transcription . This inhibition affects the transcription of several genes, including those involved in metabolic pathways .
Preparation Methods
Synthetic Routes and Reaction Conditions
The reaction conditions typically include the use of organic solvents, catalysts, and controlled temperatures to ensure the desired chemical transformations .
Industrial Production Methods
Industrial production of THZ1 Dihydrochloride follows similar synthetic routes but on a larger scale. The process involves optimizing reaction conditions to maximize yield and purity. Techniques such as crystallization and chromatography are employed to purify the final product .
Chemical Reactions Analysis
Types of Reactions
THZ1 Dihydrochloride undergoes various chemical reactions, including:
Oxidation: Involves the addition of oxygen or the removal of hydrogen.
Reduction: Involves the addition of hydrogen or the removal of oxygen.
Substitution: Involves the replacement of one functional group with another
Common Reagents and Conditions
Common reagents used in these reactions include oxidizing agents like potassium permanganate, reducing agents like sodium borohydride, and various nucleophiles for substitution reactions. The conditions often involve specific temperatures, pH levels, and solvents to facilitate the reactions .
Major Products Formed
The major products formed from these reactions depend on the specific reagents and conditions used. For example, oxidation may yield oxidized derivatives, while substitution reactions may produce various substituted analogs of this compound .
Scientific Research Applications
THZ1 Dihydrochloride has a wide range of scientific research applications, including:
Chemistry: Used as a tool compound to study the role of CDK7 in various biochemical pathways.
Biology: Employed in cell biology research to investigate the effects of CDK7 inhibition on cell cycle regulation and transcription.
Medicine: Explored as a potential therapeutic agent for treating various cancers, including non-small cell lung cancer and pancreatic cancer
Industry: Utilized in the development of new drugs targeting CDK7 and related pathways
Comparison with Similar Compounds
Similar Compounds
THZ531: A derivative of THZ1 with similar CDK7 inhibitory activity.
SY-1365: Another selective CDK7 inhibitor with potential anticancer properties.
ICEC0942 (CT7001): A CDK7 inhibitor currently in clinical trials for cancer treatment .
Uniqueness of THZ1 Dihydrochloride
This compound is unique due to its high selectivity and potency as a CDK7 inhibitor. It has demonstrated significant antiproliferative effects in various cancer cell lines and has shown potential in combination therapies with other anticancer agents .
Properties
IUPAC Name |
N-[3-[[5-chloro-4-(1H-indol-3-yl)pyrimidin-2-yl]amino]phenyl]-4-[[(E)-4-(dimethylamino)but-2-enoyl]amino]benzamide;dihydrochloride | |
---|---|---|
Details | Computed by Lexichem TK 2.7.0 (PubChem release 2021.05.07) | |
Source | PubChem | |
URL | https://pubchem.ncbi.nlm.nih.gov | |
Description | Data deposited in or computed by PubChem | |
InChI |
InChI=1S/C31H28ClN7O2.2ClH/c1-39(2)16-6-11-28(40)35-21-14-12-20(13-15-21)30(41)36-22-7-5-8-23(17-22)37-31-34-19-26(32)29(38-31)25-18-33-27-10-4-3-9-24(25)27;;/h3-15,17-19,33H,16H2,1-2H3,(H,35,40)(H,36,41)(H,34,37,38);2*1H/b11-6+;; | |
Details | Computed by InChI 1.0.6 (PubChem release 2021.05.07) | |
Source | PubChem | |
URL | https://pubchem.ncbi.nlm.nih.gov | |
Description | Data deposited in or computed by PubChem | |
InChI Key |
AJTGQOACYBCREM-QVLKBJGCSA-N | |
Details | Computed by InChI 1.0.6 (PubChem release 2021.05.07) | |
Source | PubChem | |
URL | https://pubchem.ncbi.nlm.nih.gov | |
Description | Data deposited in or computed by PubChem | |
Canonical SMILES |
CN(C)CC=CC(=O)NC1=CC=C(C=C1)C(=O)NC2=CC=CC(=C2)NC3=NC=C(C(=N3)C4=CNC5=CC=CC=C54)Cl.Cl.Cl | |
Details | Computed by OEChem 2.3.0 (PubChem release 2021.05.07) | |
Source | PubChem | |
URL | https://pubchem.ncbi.nlm.nih.gov | |
Description | Data deposited in or computed by PubChem | |
Isomeric SMILES |
CN(C)C/C=C/C(=O)NC1=CC=C(C=C1)C(=O)NC2=CC=CC(=C2)NC3=NC=C(C(=N3)C4=CNC5=CC=CC=C54)Cl.Cl.Cl | |
Details | Computed by OEChem 2.3.0 (PubChem release 2021.05.07) | |
Source | PubChem | |
URL | https://pubchem.ncbi.nlm.nih.gov | |
Description | Data deposited in or computed by PubChem | |
Molecular Formula |
C31H30Cl3N7O2 | |
Details | Computed by PubChem 2.1 (PubChem release 2021.05.07) | |
Source | PubChem | |
URL | https://pubchem.ncbi.nlm.nih.gov | |
Description | Data deposited in or computed by PubChem | |
Molecular Weight |
639.0 g/mol | |
Details | Computed by PubChem 2.1 (PubChem release 2021.05.07) | |
Source | PubChem | |
URL | https://pubchem.ncbi.nlm.nih.gov | |
Description | Data deposited in or computed by PubChem | |
Retrosynthesis Analysis
AI-Powered Synthesis Planning: Our tool employs the Template_relevance Pistachio, Template_relevance Bkms_metabolic, Template_relevance Pistachio_ringbreaker, Template_relevance Reaxys, Template_relevance Reaxys_biocatalysis model, leveraging a vast database of chemical reactions to predict feasible synthetic routes.
One-Step Synthesis Focus: Specifically designed for one-step synthesis, it provides concise and direct routes for your target compounds, streamlining the synthesis process.
Accurate Predictions: Utilizing the extensive PISTACHIO, BKMS_METABOLIC, PISTACHIO_RINGBREAKER, REAXYS, REAXYS_BIOCATALYSIS database, our tool offers high-accuracy predictions, reflecting the latest in chemical research and data.
Strategy Settings
Precursor scoring | Relevance Heuristic |
---|---|
Min. plausibility | 0.01 |
Model | Template_relevance |
Template Set | Pistachio/Bkms_metabolic/Pistachio_ringbreaker/Reaxys/Reaxys_biocatalysis |
Top-N result to add to graph | 6 |
Feasible Synthetic Routes
Disclaimer and Information on In-Vitro Research Products
Please be aware that all articles and product information presented on BenchChem are intended solely for informational purposes. The products available for purchase on BenchChem are specifically designed for in-vitro studies, which are conducted outside of living organisms. In-vitro studies, derived from the Latin term "in glass," involve experiments performed in controlled laboratory settings using cells or tissues. It is important to note that these products are not categorized as medicines or drugs, and they have not received approval from the FDA for the prevention, treatment, or cure of any medical condition, ailment, or disease. We must emphasize that any form of bodily introduction of these products into humans or animals is strictly prohibited by law. It is essential to adhere to these guidelines to ensure compliance with legal and ethical standards in research and experimentation.