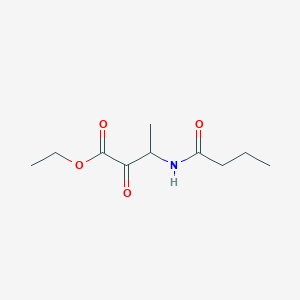
ETHYL 3-BUTANAMIDO-2-OXOBUTANOATE
Overview
Description
N-[4-(4-nitrophenylphospho)butanoyl]-D-alanine is an organic compound belonging to the class of N-acyl-alpha amino acids. This compound features a 4-(4-nitrophenylphospho)butanoyl group attached to the nitrogen of D-alanine. It is a small molecule with a molecular formula of C13H17N2O8P and a molecular weight of 360.2564 g/mol .
Preparation Methods
Synthetic Routes and Reaction Conditions
The synthesis of N-[4-(4-nitrophenylphospho)butanoyl]-D-alanine typically involves the acylation of D-alanine with 4-(4-nitrophenylphospho)butanoyl chloride. The reaction is carried out in the presence of a base, such as triethylamine, to neutralize the hydrochloric acid formed during the reaction. The reaction is usually conducted in an organic solvent like dichloromethane at low temperatures to prevent side reactions .
Industrial Production Methods
Industrial production of N-[4-(4-nitrophenylphospho)butanoyl]-D-alanine follows similar synthetic routes but on a larger scale. The process involves the use of automated reactors and continuous flow systems to ensure consistent product quality and yield. The reaction conditions are optimized to maximize the efficiency and minimize the production costs .
Chemical Reactions Analysis
Types of Reactions
N-[4-(4-nitrophenylphospho)butanoyl]-D-alanine undergoes various chemical reactions, including:
Oxidation: The nitrophenyl group can be oxidized to form nitroso or nitro derivatives.
Reduction: The nitrophenyl group can be reduced to form amino derivatives.
Substitution: The phospho group can undergo nucleophilic substitution reactions.
Common Reagents and Conditions
Oxidation: Common oxidizing agents include potassium permanganate and chromium trioxide.
Reduction: Common reducing agents include hydrogen gas with a palladium catalyst and sodium borohydride.
Substitution: Nucleophiles such as amines and alcohols can be used in substitution reactions.
Major Products Formed
Oxidation: Formation of nitroso or nitro derivatives.
Reduction: Formation of amino derivatives.
Substitution: Formation of substituted phospho derivatives.
Scientific Research Applications
N-[4-(4-nitrophenylphospho)butanoyl]-D-alanine has several scientific research applications:
Chemistry: Used as a building block in the synthesis of more complex molecules.
Biology: Studied for its potential role in enzyme inhibition and protein modification.
Medicine: Investigated for its potential therapeutic effects, including anti-inflammatory and anticancer properties.
Industry: Used in the development of novel materials and as a reagent in various chemical processes.
Mechanism of Action
The mechanism of action of N-[4-(4-nitrophenylphospho)butanoyl]-D-alanine involves its interaction with specific molecular targets, such as enzymes and receptors. The compound can inhibit enzyme activity by binding to the active site or allosteric site, thereby preventing substrate binding and catalysis. The nitrophenyl group can also interact with cellular receptors, modulating signal transduction pathways and cellular responses .
Comparison with Similar Compounds
Similar Compounds
N-[4-(4-nitrophenylphospho)butanoyl]-L-alanine: An enantiomer of N-[4-(4-nitrophenylphospho)butanoyl]-D-alanine with similar chemical properties but different biological activities.
N-acyl-alpha amino acids: A class of compounds with similar structural features but varying acyl groups and amino acids.
Uniqueness
N-[4-(4-nitrophenylphospho)butanoyl]-D-alanine is unique due to its specific combination of a nitrophenylphospho group and D-alanine. This unique structure imparts distinct chemical reactivity and biological activity, making it valuable for various scientific research applications .
Properties
IUPAC Name |
ethyl 3-(butanoylamino)-2-oxobutanoate | |
---|---|---|
Source | PubChem | |
URL | https://pubchem.ncbi.nlm.nih.gov | |
Description | Data deposited in or computed by PubChem | |
InChI |
InChI=1S/C10H17NO4/c1-4-6-8(12)11-7(3)9(13)10(14)15-5-2/h7H,4-6H2,1-3H3,(H,11,12) | |
Source | PubChem | |
URL | https://pubchem.ncbi.nlm.nih.gov | |
Description | Data deposited in or computed by PubChem | |
InChI Key |
CEHSJTHDBNDHMT-UHFFFAOYSA-N | |
Source | PubChem | |
URL | https://pubchem.ncbi.nlm.nih.gov | |
Description | Data deposited in or computed by PubChem | |
Canonical SMILES |
CCCC(=O)NC(C)C(=O)C(=O)OCC | |
Source | PubChem | |
URL | https://pubchem.ncbi.nlm.nih.gov | |
Description | Data deposited in or computed by PubChem | |
Molecular Formula |
C10H17NO4 | |
Source | PubChem | |
URL | https://pubchem.ncbi.nlm.nih.gov | |
Description | Data deposited in or computed by PubChem | |
Molecular Weight |
215.25 g/mol | |
Source | PubChem | |
URL | https://pubchem.ncbi.nlm.nih.gov | |
Description | Data deposited in or computed by PubChem | |
Synthesis routes and methods I
Procedure details
Synthesis routes and methods II
Procedure details
Disclaimer and Information on In-Vitro Research Products
Please be aware that all articles and product information presented on BenchChem are intended solely for informational purposes. The products available for purchase on BenchChem are specifically designed for in-vitro studies, which are conducted outside of living organisms. In-vitro studies, derived from the Latin term "in glass," involve experiments performed in controlled laboratory settings using cells or tissues. It is important to note that these products are not categorized as medicines or drugs, and they have not received approval from the FDA for the prevention, treatment, or cure of any medical condition, ailment, or disease. We must emphasize that any form of bodily introduction of these products into humans or animals is strictly prohibited by law. It is essential to adhere to these guidelines to ensure compliance with legal and ethical standards in research and experimentation.