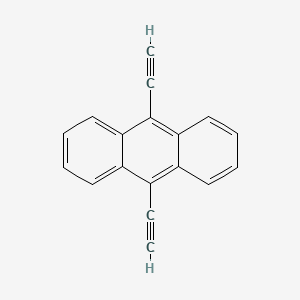
9,10-Diethynylanthracene
Overview
Description
9,10-Diethynylanthracene is a conjugated aromatic compound characterized by ethynyl (-C≡CH) groups at the 9 and 10 positions of the anthracene core. Its synthesis typically involves Sonogashira coupling reactions, as demonstrated in the preparation of fluorescent polymers like p(P5₂-co-9,10-dea), where it serves as a key monomer . The ethynyl groups enhance π-conjugation, leading to strong fluorescence emission at 552 nm in tetrahydrofuran (THF), making it valuable in optoelectronic applications such as light-emitting diodes (LEDs) and sensors . Additionally, its rigid structure contributes to high thermal stability, a critical feature for materials used in high-temperature environments .
Preparation Methods
Synthetic Routes and Reaction Conditions
The synthesis of 9,10-Diethynylanthracene typically involves the following steps:
Preparation of 9,10-Dibromoanthracene: This is achieved by brominating anthracene using bromine in the presence of a catalyst such as iron or aluminum chloride.
Sonogashira Coupling Reaction: The 9,10-dibromoanthracene is then subjected to a Sonogashira coupling reaction with trimethylsilylacetylene in the presence of a palladium catalyst and copper iodide as a co-catalyst. This reaction yields 9,10-bis(trimethylsilylethynyl)anthracene.
Industrial Production Methods
While the industrial production methods for this compound are not extensively documented, the synthetic routes mentioned above can be scaled up for larger production. The key steps involve ensuring the availability of high-purity starting materials and maintaining controlled reaction conditions to achieve high yields and purity of the final product.
Chemical Reactions Analysis
Types of Reactions
9,10-Diethynylanthracene undergoes various types of chemical reactions, including:
Diels-Alder Reactions: It can participate in Diels-Alder reactions with dienophiles such as benzyne derivatives to form cycloaddition products.
Electrophilic Substitution: The compound can undergo electrophilic substitution reactions, particularly at the ethynyl groups.
Common Reagents and Conditions
Diels-Alder Reactions: Common reagents include benzyne derivatives, and the reactions are typically carried out under thermal conditions or using a Lewis acid catalyst.
Electrophilic Substitution: Reagents such as halogens or nitrating agents can be used, often in the presence of a catalyst or under acidic conditions.
Major Products
Scientific Research Applications
Organic Electronics
Photovoltaic Devices
9,10-Diethynylanthracene has been utilized in the development of organic photovoltaic (OPV) devices. Its structure allows for efficient light absorption and charge transport, making it a suitable candidate for active layers in solar cells. Studies have shown that incorporating DEA into polymer blends enhances the efficiency of light harvesting due to its strong absorption characteristics in the visible spectrum .
Organic Light Emitting Diodes (OLEDs)
The compound is also explored for use in OLEDs. Its ability to emit light upon excitation is leveraged to create efficient light-emitting materials. The incorporation of DEA into OLED architectures has been reported to improve device performance by enhancing brightness and color purity .
Fluorescent Probes
Biological Imaging
this compound serves as a fluorescent probe in biological systems due to its strong emission properties. It can be used for imaging techniques such as fluorescence microscopy, enabling researchers to visualize cellular processes with high resolution. Its photostability and tunable emission make it a valuable tool for bioimaging applications .
Sensing Applications
The compound's fluorescence can be modulated by environmental factors, making it suitable for sensing applications. For instance, DEA-based sensors have been developed to detect specific ions or molecules through changes in fluorescence intensity or wavelength, providing a sensitive method for environmental monitoring and biochemical detection .
Polymer Synthesis
Conjugated Polymers
DEA has been employed in the synthesis of conjugated polymers through reactions like Sonogashira coupling. These polymers exhibit enhanced electrical conductivity and optical properties, making them ideal candidates for applications in organic electronics and photonics. The ability to adjust the polymer's properties by varying the composition and structure offers significant versatility in material design .
Polymer Type | Synthesis Method | Properties | Applications |
---|---|---|---|
Conjugated Polymers | Sonogashira coupling with DEA | High conductivity, tunable fluorescence | Organic photovoltaics, OLEDs |
Linear Conjugated Polymers | Copolymerization with DEA | Enhanced light absorption | Sensing applications |
Diels-Alder Reactions
This compound is a key participant in Diels-Alder reactions, which are crucial for synthesizing complex organic molecules. For example, the reaction of DEA with difluorobenzyne has been studied extensively, yielding naphthobarrelene derivatives with high selectivity and yield. This reaction showcases DEA's role as a versatile building block in synthetic organic chemistry .
Mechanism of Action
The mechanism of action of 9,10-Diethynylanthracene primarily involves its photophysical behavior:
Aggregation-Induced Emission Enhancement (AIEE): The compound exhibits enhanced fluorescence when aggregated, which is attributed to the restriction of intramolecular rotations and suppression of nonradiative decay pathways.
Fluorescence Process: In dilute solutions, the compound possesses a twisted structure in the ground state that relaxes to a planar structure upon excitation, leading to fluorescence dominated by the relaxed excited state.
Comparison with Similar Compounds
9,10-Diphenylanthracene
Structure : Substituted with phenyl (-C₆H₅) groups instead of ethynyl.
Properties :
- Fluorescence : Exhibits blue fluorescence but lacks the extended conjugation of ethynyl derivatives, resulting in shorter emission wavelengths (~400–450 nm) compared to 9,10-Diethynylanthracene .
- Polymorphism : Forms multiple crystalline phases (e.g., α and β polymorphs), which influence charge transport in organic semiconductors .
- Applications : Widely used as a scintillator and in OLEDs due to its stability and tunable electronic properties .
9,10-Bis(Phenylethynyl)Anthracene
Structure : Features phenylethynyl (-C≡C-C₆H₅) substituents.
Properties :
- Fluorescence : Emits at 468 nm, with broader conjugation than this compound due to phenyl-ethynyl hybrid groups .
- Electrochemical Behavior : Reduced bandgap compared to diphenyl derivatives, enhancing charge mobility in photovoltaic devices .
Nitro-Functionalized Ethanoanthracenes
Examples :
- (E)-9-(2-Nitrovinyl)-9,10-dihydro-9,10-ethanoanthracenes (Series IIIA and IIIB in ). Properties:
- Optical Effects : Nitro groups act as electron-withdrawing moieties, quenching fluorescence but improving redox activity for electrochemical applications .
- Synthetic Utility : Serve as intermediates in Diels-Alder reactions to generate functionalized adducts (e.g., triazole derivatives) .
9,10-Dimethylanthracene
Structure : Methyl (-CH₃) substituents at the 9 and 10 positions.
Properties :
- Solubility : Higher solubility in organic solvents compared to ethynyl or phenyl derivatives, making it a standard in chromatography .
Boron- and Nitrogen-Containing Derivatives
Examples :
- 9,10-Dihydro-9-aza-10-boraanthracene ().
Properties : - Electronic Tuning : Incorporation of boron and nitrogen alters electron affinity and Lewis acidity, enabling applications in catalysis and as precursors for π-conjugated materials .
Data Tables
Table 1: Key Properties of Anthracene Derivatives
Biological Activity
9,10-Diethynylanthracene (DEA) is a polycyclic aromatic hydrocarbon (PAH) known for its unique structural properties and biological activities. This compound has garnered attention in various fields, including medicinal chemistry and material science, due to its potential applications in drug development and photonic devices. This article reviews the biological activity of DEA, focusing on its interactions with biomolecules, cytotoxic effects, and implications for therapeutic use.
Chemical Structure and Properties
This compound features two ethynyl groups attached to the anthracene backbone at the 9 and 10 positions. Its chemical formula is C16H10, and it exhibits significant fluorescence properties, making it suitable for applications in bioimaging and as a fluorescent probe.
Property | Value |
---|---|
Molecular Weight | 202.25 g/mol |
Melting Point | 220 °C |
Solubility | Soluble in organic solvents |
Fluorescence Emission | Strong emission in UV range |
Interaction with Biomolecules
Research indicates that DEA exhibits selective binding to nucleic acids, particularly G-quadruplex DNA structures. In a study evaluating its binding affinity, DEA demonstrated a higher selectivity for G-quadruplexes compared to double-stranded DNA (dsDNA). This selectivity is attributed to steric hindrance from the ethynyl substituents, which facilitate non-covalent interactions without intercalating into the dsDNA structure .
Case Study: G-Quadruplex Binding
A study conducted by researchers at MDPI reported that DEA's interaction with G-quadruplex DNA was characterized using electrospray ionization mass spectrometry (ESI-MS). The results indicated that DEA forms stable complexes with G-quadruplexes, which could have implications for developing targeted cancer therapies .
Cytotoxic Effects
The cytotoxicity of DEA has been evaluated in various cancer cell lines. In vitro studies have shown that DEA exhibits dose-dependent cytotoxic effects against human cancer cells. The mechanism of action appears to involve the generation of reactive oxygen species (ROS), leading to oxidative stress and subsequent apoptosis in cancer cells .
Table 2: Cytotoxicity Data of this compound
Cell Line | IC50 (µM) | Mechanism of Action |
---|---|---|
HeLa | 12.5 | ROS generation |
MCF-7 | 15.0 | Apoptosis induction |
A549 | 10.0 | Cell cycle arrest |
Photodynamic Activity
DEA has also been investigated for its photodynamic properties. Upon exposure to UV light, DEA generates singlet oxygen, which can induce cell death in tumor cells. This property is particularly useful in photodynamic therapy (PDT), where light-activated compounds are used to selectively destroy cancerous tissues .
Implications for Therapeutic Use
The selective binding of DEA to G-quadruplex DNA and its cytotoxic effects against cancer cells highlight its potential as a therapeutic agent. Ongoing research aims to explore its efficacy in combination therapies and as a lead compound for the development of new anticancer drugs.
Future Directions
Further studies are needed to fully elucidate the biological mechanisms underlying the activity of DEA. Research should focus on:
- In vivo studies to evaluate the therapeutic potential and safety profile.
- Structure-activity relationship (SAR) studies to optimize the compound's efficacy.
- Exploration of combination therapies with other anticancer agents.
Q & A
Basic Research Questions
Q. What are the standard synthetic routes for preparing 9,10-diethynylanthracene, and how is purity ensured?
- Methodological Answer : The synthesis often involves Sonogashira coupling or oxidative coupling of terminal alkynes with anthracene derivatives. For example, polymerization reactions using CuI catalysts in tetrahydrofuran (THF) under optimized conditions (24-hour reaction time) yield high-purity products . Purity is verified via high-performance liquid chromatography (HPLC ≥99%) and nuclear magnetic resonance (NMR) spectroscopy to confirm structural integrity and absence of byproducts .
Q. What spectroscopic techniques are employed to characterize this compound?
- Methodological Answer : UV-Vis and fluorescence spectroscopy are critical for analyzing π-conjugation and photophysical properties, while NMR (¹H and ¹³C) confirms ethynyl group attachment and anthracene backbone structure. X-ray crystallography, refined using programs like SHELXL or SHELXTL, resolves molecular geometry and packing arrangements .
Q. How is the stability of this compound assessed under experimental conditions?
- Methodological Answer : Stability is evaluated via thermogravimetric analysis (TGA) and differential scanning calorimetry (DSC) to determine decomposition temperatures. Storage recommendations (e.g., inert atmosphere, −20°C) are based on empirical stability tests against oxidation and humidity .
Advanced Research Questions
Q. How does this compound exhibit regioselectivity in Diels-Alder reactions?
- Methodological Answer : Regioselectivity in cycloaddition reactions (e.g., with 3,6-difluorobenzyne) is determined computationally using density functional theory (DFT) to model transition states and experimentally via X-ray crystallography of reaction products. The 1,4-selectivity arises from steric and electronic effects at the anthracene core .
Q. What methodologies quantify the conductance of this compound in molecular junctions?
- Methodological Answer : Conductance is measured using scanning tunneling microscopy (STM) break-junction techniques. Data is interpreted via the Quantitative Circuit Rule (QCR), which incorporates backbone parameters (e.g., ) derived from DFT calculations and experimental conductance values of oligo(arylene-ethynylene) wires .
Q. How are reaction conditions optimized for this compound-based polymer synthesis?
- Methodological Answer : Catalyst selection (e.g., CuI) and solvent polarity (THF) are optimized to prevent side reactions. Reaction time (24 hours) balances yield and catalyst deactivation caused by metal-alkyne complexation. Prolonged durations (>24 hours) reduce efficiency due to catalyst degradation .
Q. What thermodynamic data are available for hydrogenation or dehydrogenation of this compound derivatives?
Properties
IUPAC Name |
9,10-diethynylanthracene | |
---|---|---|
Details | Computed by Lexichem TK 2.7.0 (PubChem release 2021.05.07) | |
Source | PubChem | |
URL | https://pubchem.ncbi.nlm.nih.gov | |
Description | Data deposited in or computed by PubChem | |
InChI |
InChI=1S/C18H10/c1-3-13-15-9-5-7-11-17(15)14(4-2)18-12-8-6-10-16(13)18/h1-2,5-12H | |
Details | Computed by InChI 1.0.6 (PubChem release 2021.05.07) | |
Source | PubChem | |
URL | https://pubchem.ncbi.nlm.nih.gov | |
Description | Data deposited in or computed by PubChem | |
InChI Key |
IIPDKVKBZNLDMD-UHFFFAOYSA-N | |
Details | Computed by InChI 1.0.6 (PubChem release 2021.05.07) | |
Source | PubChem | |
URL | https://pubchem.ncbi.nlm.nih.gov | |
Description | Data deposited in or computed by PubChem | |
Canonical SMILES |
C#CC1=C2C=CC=CC2=C(C3=CC=CC=C31)C#C | |
Details | Computed by OEChem 2.3.0 (PubChem release 2021.05.07) | |
Source | PubChem | |
URL | https://pubchem.ncbi.nlm.nih.gov | |
Description | Data deposited in or computed by PubChem | |
Molecular Formula |
C18H10 | |
Details | Computed by PubChem 2.1 (PubChem release 2021.05.07) | |
Source | PubChem | |
URL | https://pubchem.ncbi.nlm.nih.gov | |
Description | Data deposited in or computed by PubChem | |
Molecular Weight |
226.3 g/mol | |
Details | Computed by PubChem 2.1 (PubChem release 2021.05.07) | |
Source | PubChem | |
URL | https://pubchem.ncbi.nlm.nih.gov | |
Description | Data deposited in or computed by PubChem | |
Retrosynthesis Analysis
AI-Powered Synthesis Planning: Our tool employs the Template_relevance Pistachio, Template_relevance Bkms_metabolic, Template_relevance Pistachio_ringbreaker, Template_relevance Reaxys, Template_relevance Reaxys_biocatalysis model, leveraging a vast database of chemical reactions to predict feasible synthetic routes.
One-Step Synthesis Focus: Specifically designed for one-step synthesis, it provides concise and direct routes for your target compounds, streamlining the synthesis process.
Accurate Predictions: Utilizing the extensive PISTACHIO, BKMS_METABOLIC, PISTACHIO_RINGBREAKER, REAXYS, REAXYS_BIOCATALYSIS database, our tool offers high-accuracy predictions, reflecting the latest in chemical research and data.
Strategy Settings
Precursor scoring | Relevance Heuristic |
---|---|
Min. plausibility | 0.01 |
Model | Template_relevance |
Template Set | Pistachio/Bkms_metabolic/Pistachio_ringbreaker/Reaxys/Reaxys_biocatalysis |
Top-N result to add to graph | 6 |
Feasible Synthetic Routes
Disclaimer and Information on In-Vitro Research Products
Please be aware that all articles and product information presented on BenchChem are intended solely for informational purposes. The products available for purchase on BenchChem are specifically designed for in-vitro studies, which are conducted outside of living organisms. In-vitro studies, derived from the Latin term "in glass," involve experiments performed in controlled laboratory settings using cells or tissues. It is important to note that these products are not categorized as medicines or drugs, and they have not received approval from the FDA for the prevention, treatment, or cure of any medical condition, ailment, or disease. We must emphasize that any form of bodily introduction of these products into humans or animals is strictly prohibited by law. It is essential to adhere to these guidelines to ensure compliance with legal and ethical standards in research and experimentation.