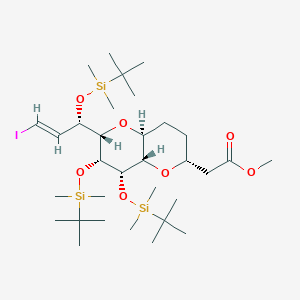
Eribulin mesylate intermediate
Overview
Description
Eribulin mesylate intermediate is a crucial compound in the synthesis of eribulin mesylate, a synthetic analogue of halichondrin B. Halichondrin B is a natural product isolated from the marine sponge Halichondria okadai. Eribulin mesylate is primarily used as an anti-cancer agent, particularly in the treatment of metastatic breast cancer and liposarcoma .
Mechanism of Action
Target of Action
Eribulin mesylate, also known as “Methyl 2-((2R,4aS,6S,7R,8S,8aS)-7,8-bis((tert-butyldimethylsilyl)oxy)-6-((S,E)-1-((tert-butyldimethylsilyl)oxy)-3-iodoallyl)octahydropyrano[3,2-b]pyran-2-yl)acetate”, primarily targets microtubules . Microtubules are a component of the cell’s cytoskeleton and play a crucial role in maintaining cell shape, enabling cell motility, and most importantly, in cell division .
Mode of Action
Eribulin inhibits the growth phase of microtubules without affecting the shortening phase, leading to the sequestration of tubulin into nonproductive aggregates . This unique mechanism disrupts the normal dynamic reorganization of the microtubule network that is essential for interphase and mitotic cellular functions . The disruption leads to a G2/M cell-cycle block, disruption of mitotic spindles, and ultimately, apoptotic cell death after prolonged mitotic blockage .
Biochemical Pathways
Eribulin’s action on microtubules affects multiple biochemical pathways. It disrupts normal cell division, leading to cell cycle arrest at the G2/M phase . This disruption can lead to apoptosis, or programmed cell death . Additionally, eribulin has been observed to have effects on peripheral nerves, angiogenesis, vascular remodeling, and epithelial-to-mesenchymal transition .
Result of Action
The primary result of eribulin’s action is the induction of cell cycle arrest and subsequent apoptotic cell death . This leads to a reduction in the proliferation of cancer cells, thereby inhibiting tumor growth . Clinical trial results have demonstrated that eribulin treatment provides a survival advantage to patients with metastatic or locally advanced breast cancer previously treated with an anthracycline and a taxane .
Action Environment
The action of eribulin can be influenced by various environmental factors. For instance, the presence of other drugs could potentially affect its metabolism and excretion . Furthermore, patient-specific factors such as renal function can impact the drug’s pharmacokinetics and overall effectiveness . Therefore, careful consideration of these factors is necessary when administering eribulin to ensure optimal therapeutic outcomes.
Biochemical Analysis
Biochemical Properties
Eribulin mesylate intermediate plays a crucial role in biochemical reactions, particularly in the inhibition of microtubule polymerization. This compound interacts with tubulin, a protein that is essential for microtubule formation. By binding to tubulin, this compound prevents the polymerization of microtubules, leading to cell cycle arrest and apoptosis . The interaction between this compound and tubulin is highly specific and results in the sequestration of tubulin into nonproductive aggregates .
Cellular Effects
This compound has significant effects on various types of cells and cellular processes. It influences cell function by disrupting the mitotic spindle formation, leading to cell cycle arrest at the G2/M phase . This disruption results in prolonged mitotic blockage and ultimately induces apoptotic cell death . Additionally, this compound affects cell signaling pathways, gene expression, and cellular metabolism by altering the dynamics of microtubules .
Molecular Mechanism
The molecular mechanism of this compound involves its binding to the plus ends of microtubules, inhibiting their growth while allowing the shortening phase to continue . This unique mechanism of action distinguishes this compound from other microtubule-targeting agents. By preventing microtubule polymerization, this compound induces cell cycle arrest and apoptosis through the activation of various signaling pathways and changes in gene expression .
Temporal Effects in Laboratory Settings
In laboratory settings, the effects of this compound change over time. The compound exhibits stability under controlled conditions, but its degradation can occur under certain environmental factors . Long-term studies have shown that this compound maintains its inhibitory effects on microtubule polymerization and continues to induce cell cycle arrest and apoptosis in both in vitro and in vivo models .
Dosage Effects in Animal Models
The effects of this compound vary with different dosages in animal models. At lower doses, the compound effectively inhibits tumor growth and induces apoptosis without causing significant toxicity . At higher doses, this compound can lead to adverse effects such as neutropenia and fatigue . These dosage-dependent effects highlight the importance of optimizing the dosage for therapeutic efficacy while minimizing toxicity.
Metabolic Pathways
This compound is involved in metabolic pathways that include interactions with enzymes such as CYP3A4 . Although metabolism represents a minor component in the clearance of this compound, it is essential to consider its effects on metabolic flux and metabolite levels . The compound’s interaction with CYP3A4 does not significantly affect its clearance, indicating a relatively stable metabolic profile .
Transport and Distribution
The transport and distribution of this compound within cells and tissues involve rapid distribution and slow-to-moderate clearance . The compound is primarily eliminated through feces, with a half-life of approximately 40 hours . The distribution of this compound is dose-dependent, with higher doses achieving higher peak plasma concentrations .
Subcellular Localization
This compound localizes primarily to the cytoplasm, where it exerts its inhibitory effects on microtubule polymerization . The compound’s activity is influenced by its subcellular localization, as it targets the microtubules within the cytoplasm . Post-translational modifications and targeting signals may also play a role in directing this compound to specific cellular compartments .
Preparation Methods
Synthetic Routes and Reaction Conditions
The preparation of eribulin mesylate intermediate involves several steps:
-
De-protection of Eribulin-Enone: : The process begins with the de-protection of eribulin-enone in tetrahydrofuran using a TBAF solution buffered with imidazole hydrochloride in the presence of molecular sieves and sodium sulfate. This results in an in-situ mixture of eribulin-dione diastereomers at the C12 carbon .
-
Ketalization: : The in-situ mixture of eribulin-dione diastereomers undergoes ketalization with PPTS in dichloromethane to yield eribulin-diol .
-
Tosylation: : The eribulin-diol is then tosylated using para-toluene sulfonic anhydride in the presence of 2,4,6-collidine, producing eribulin-tosylate .
-
Amination: : The resulting eribulin-tosylate is aminated using ammonium hydroxide in isopropyl alcohol to obtain eribulin base .
-
Formation of Eribulin Mesylate: : Finally, the eribulin base is treated with methanesulfonic acid in an acetonitrile and water mixture to produce eribulin mesylate .
Industrial Production Methods
The industrial production of this compound follows similar synthetic routes but is optimized for large-scale production. This involves the use of automated reactors, precise control of reaction conditions, and efficient purification techniques to ensure high yield and purity of the intermediate .
Chemical Reactions Analysis
Types of Reactions
Eribulin mesylate intermediate undergoes various chemical reactions, including:
Oxidation: The intermediate can be oxidized to form different oxidation states, which are crucial for subsequent reactions.
Reduction: Reduction reactions are used to modify the functional groups and achieve the desired stereochemistry.
Substitution: Substitution reactions are employed to introduce or replace functional groups, enhancing the compound’s reactivity and stability.
Common Reagents and Conditions
Oxidation: Common oxidizing agents include potassium permanganate and chromium trioxide.
Reduction: Reducing agents such as sodium borohydride and lithium aluminum hydride are frequently used.
Substitution: Reagents like halides, sulfonates, and amines are used under various conditions, including acidic or basic environments.
Major Products Formed
The major products formed from these reactions include various diastereomers, ketals, tosylates, and the final eribulin mesylate compound .
Scientific Research Applications
Eribulin mesylate intermediate has numerous applications in scientific research:
Chemistry: It is used in the study of complex organic synthesis and the development of new synthetic methodologies.
Biology: The compound is studied for its interactions with biological molecules, particularly tubulin, which is crucial for cell division.
Comparison with Similar Compounds
Similar Compounds
Paclitaxel: Another microtubule-targeting agent used in cancer treatment.
Docetaxel: Similar to paclitaxel, it stabilizes microtubules and prevents their depolymerization.
Ixabepilone: A microtubule-stabilizing agent used in the treatment of metastatic breast cancer.
Uniqueness of Eribulin Mesylate
Eribulin mesylate is unique due to its distinct mechanism of action. Unlike other microtubule-targeting agents, it inhibits the growth phase of microtubules without affecting the shortening phase, leading to a unique pattern of microtubule dynamics disruption . This results in a different spectrum of antitumor activity and side effects, making it a valuable addition to the arsenal of anticancer agents .
Properties
IUPAC Name |
methyl 2-[(2S,3R,4S,4aS,6R,8aS)-3,4-bis[[tert-butyl(dimethyl)silyl]oxy]-2-[(E,1S)-1-[tert-butyl(dimethyl)silyl]oxy-3-iodoprop-2-enyl]-2,3,4,4a,6,7,8,8a-octahydropyrano[3,2-b]pyran-6-yl]acetate | |
---|---|---|
Details | Computed by LexiChem 2.6.6 (PubChem release 2019.06.18) | |
Source | PubChem | |
URL | https://pubchem.ncbi.nlm.nih.gov | |
Description | Data deposited in or computed by PubChem | |
InChI |
InChI=1S/C32H63IO7Si3/c1-30(2,3)41(11,12)38-24(19-20-33)27-29(40-43(15,16)32(7,8)9)28(39-42(13,14)31(4,5)6)26-23(37-27)18-17-22(36-26)21-25(34)35-10/h19-20,22-24,26-29H,17-18,21H2,1-16H3/b20-19+/t22-,23+,24+,26+,27+,28+,29-/m1/s1 | |
Details | Computed by InChI 1.0.5 (PubChem release 2019.06.18) | |
Source | PubChem | |
URL | https://pubchem.ncbi.nlm.nih.gov | |
Description | Data deposited in or computed by PubChem | |
InChI Key |
MIEJSEPCTMXSCI-SUCSLEJPSA-N | |
Details | Computed by InChI 1.0.5 (PubChem release 2019.06.18) | |
Source | PubChem | |
URL | https://pubchem.ncbi.nlm.nih.gov | |
Description | Data deposited in or computed by PubChem | |
Canonical SMILES |
CC(C)(C)[Si](C)(C)OC1C2C(CCC(O2)CC(=O)OC)OC(C1O[Si](C)(C)C(C)(C)C)C(C=CI)O[Si](C)(C)C(C)(C)C | |
Details | Computed by OEChem 2.1.5 (PubChem release 2019.06.18) | |
Source | PubChem | |
URL | https://pubchem.ncbi.nlm.nih.gov | |
Description | Data deposited in or computed by PubChem | |
Isomeric SMILES |
CC(C)(C)[Si](C)(C)O[C@H]1[C@@H]2[C@H](CC[C@@H](O2)CC(=O)OC)O[C@H]([C@H]1O[Si](C)(C)C(C)(C)C)[C@H](/C=C/I)O[Si](C)(C)C(C)(C)C | |
Details | Computed by OEChem 2.1.5 (PubChem release 2019.06.18) | |
Source | PubChem | |
URL | https://pubchem.ncbi.nlm.nih.gov | |
Description | Data deposited in or computed by PubChem | |
Molecular Formula |
C32H63IO7Si3 | |
Details | Computed by PubChem 2.1 (PubChem release 2019.06.18) | |
Source | PubChem | |
URL | https://pubchem.ncbi.nlm.nih.gov | |
Description | Data deposited in or computed by PubChem | |
Molecular Weight |
771.0 g/mol | |
Details | Computed by PubChem 2.1 (PubChem release 2021.05.07) | |
Source | PubChem | |
URL | https://pubchem.ncbi.nlm.nih.gov | |
Description | Data deposited in or computed by PubChem | |
Retrosynthesis Analysis
AI-Powered Synthesis Planning: Our tool employs the Template_relevance Pistachio, Template_relevance Bkms_metabolic, Template_relevance Pistachio_ringbreaker, Template_relevance Reaxys, Template_relevance Reaxys_biocatalysis model, leveraging a vast database of chemical reactions to predict feasible synthetic routes.
One-Step Synthesis Focus: Specifically designed for one-step synthesis, it provides concise and direct routes for your target compounds, streamlining the synthesis process.
Accurate Predictions: Utilizing the extensive PISTACHIO, BKMS_METABOLIC, PISTACHIO_RINGBREAKER, REAXYS, REAXYS_BIOCATALYSIS database, our tool offers high-accuracy predictions, reflecting the latest in chemical research and data.
Strategy Settings
Precursor scoring | Relevance Heuristic |
---|---|
Min. plausibility | 0.01 |
Model | Template_relevance |
Template Set | Pistachio/Bkms_metabolic/Pistachio_ringbreaker/Reaxys/Reaxys_biocatalysis |
Top-N result to add to graph | 6 |
Feasible Synthetic Routes
Disclaimer and Information on In-Vitro Research Products
Please be aware that all articles and product information presented on BenchChem are intended solely for informational purposes. The products available for purchase on BenchChem are specifically designed for in-vitro studies, which are conducted outside of living organisms. In-vitro studies, derived from the Latin term "in glass," involve experiments performed in controlled laboratory settings using cells or tissues. It is important to note that these products are not categorized as medicines or drugs, and they have not received approval from the FDA for the prevention, treatment, or cure of any medical condition, ailment, or disease. We must emphasize that any form of bodily introduction of these products into humans or animals is strictly prohibited by law. It is essential to adhere to these guidelines to ensure compliance with legal and ethical standards in research and experimentation.