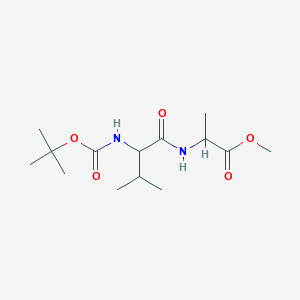
BOC-Val-Ala-OMe
Overview
Description
BOC-Val-Ala-OMe, also known as tert-butoxycarbonyl-valine-alanine-methyl ester, is a dipeptide derivative. It is commonly used in peptide synthesis and research due to its stability and ease of handling. The compound consists of valine and alanine amino acids, with protective groups that prevent unwanted reactions during synthesis.
Mechanism of Action
Target of Action
BOC-Val-Ala-OMe is a dipeptide that exhibits sequence homogeneity with the C-terminus of Alzheimer’s Aβ 39–40 and Aβ 41–42 . The primary targets of this compound are the β-sheet structures in these proteins .
Mode of Action
This compound interacts with its targets by forming intermolecular hydrogen-bonded supramolecular parallel β-sheet structures in crystalline form . This interaction results in the formation of highly organized structures .
Biochemical Pathways
The interaction of this compound with its targets affects the biochemical pathways related to the formation of β-sheet structures in Alzheimer’s Aβ 39–40 and Aβ 41–42 . The downstream effects of this interaction include the formation of highly organized structures .
Result of Action
The result of this compound’s action is the formation of highly organized structures . These structures are found to bind with the amyloid binding dyes thioflavin T (ThT) and Congo red .
Action Environment
The action of this compound is influenced by environmental factors such as the solvent used. For example, in a methanol–water solvent mixture, this compound forms a hollow hexagonal tube-like structure . The efficacy and stability of this compound are likely to be influenced by similar environmental factors.
Biochemical Analysis
Biochemical Properties
BOC-Val-Ala-OMe plays a significant role in biochemical reactions, particularly in the context of peptide synthesis. It is known to interact with lysosomal proteolytic enzymes, which effectively cleave the Val-Ala bond . This interaction is crucial for the design of antibody-drug conjugates (ADCs) where this compound serves as a cleavable linker. The stability of this compound in human plasma makes it a potent strategy in ADC linker design . Additionally, the compound exhibits intermolecular hydrogen-bonded supramolecular parallel β-sheet structures in crystalline form .
Cellular Effects
This compound influences various cellular processes, including cell signaling pathways, gene expression, and cellular metabolism. The compound’s ability to form β-sheet structures suggests its potential role in amyloidogenesis, which is relevant to neurodegenerative diseases like Alzheimer’s . Furthermore, this compound has been shown to bind with amyloid binding dyes such as thioflavin T and Congo red, indicating its involvement in amyloid fibril formation .
Molecular Mechanism
At the molecular level, this compound exerts its effects through specific binding interactions with biomolecules. The compound’s structure allows it to participate in hydrogen bonding, which is essential for its role in forming β-sheet structures . These interactions are crucial for its function in peptide synthesis and protein folding. Additionally, this compound can be deprotected under mild acidic conditions to form the free amine, which further interacts with various enzymes and proteins .
Temporal Effects in Laboratory Settings
In laboratory settings, the effects of this compound change over time due to its stability and degradation properties. The compound is stable in human plasma, which is advantageous for long-term studies . Its degradation in different solvent mixtures can lead to the formation of distinct supramolecular structures . These changes can impact its interactions with cellular components and its overall biochemical activity.
Dosage Effects in Animal Models
The effects of this compound vary with different dosages in animal models. At lower doses, the compound effectively interacts with target enzymes and proteins without causing significant adverse effects. At higher doses, this compound may exhibit toxic effects, including disruption of cellular processes and potential cytotoxicity . These dosage-dependent effects are crucial for determining the therapeutic window and safety profile of the compound.
Metabolic Pathways
This compound is involved in metabolic pathways that include interactions with lysosomal proteolytic enzymes . These enzymes cleave the Val-Ala bond, releasing the active components of the compound. The metabolic stability of this compound in human plasma ensures its prolonged activity and effectiveness in biochemical applications . Additionally, the compound’s ability to form β-sheet structures may influence metabolic flux and metabolite levels .
Transport and Distribution
Within cells and tissues, this compound is transported and distributed through interactions with specific transporters and binding proteins. These interactions facilitate its localization and accumulation in target compartments . The compound’s stability in human plasma also contributes to its effective distribution in vivo .
Subcellular Localization
This compound exhibits specific subcellular localization, which is influenced by its structural properties and interactions with cellular components. The compound’s ability to form β-sheet structures directs it to specific compartments, such as lysosomes, where it interacts with proteolytic enzymes . Additionally, post-translational modifications and targeting signals may further influence its subcellular localization and activity.
Preparation Methods
Synthetic Routes and Reaction Conditions
BOC-Val-Ala-OMe is typically synthesized through a stepwise process involving the protection of amino groups and the formation of peptide bonds. The synthesis begins with the protection of the amino group of valine using tert-butoxycarbonyl (BOC) group. This is followed by coupling the protected valine with alanine methyl ester using a coupling reagent such as dicyclohexylcarbodiimide (DCC) in the presence of a base like N,N-diisopropylethylamine (DIPEA). The reaction is carried out in an organic solvent like dichloromethane (DCM) at room temperature .
Industrial Production Methods
Industrial production of this compound follows similar synthetic routes but on a larger scale. The process involves the use of automated peptide synthesizers and large-scale reactors to ensure high yield and purity. Solvent-free synthesis methods, such as ball-milling, have also been explored to reduce environmental impact and improve efficiency .
Chemical Reactions Analysis
Types of Reactions
BOC-Val-Ala-OMe undergoes various chemical reactions, including:
Hydrolysis: The ester group can be hydrolyzed to form the corresponding carboxylic acid.
Deprotection: The BOC group can be removed under acidic conditions to yield the free amine.
Coupling Reactions: It can participate in peptide bond formation with other amino acids or peptides.
Common Reagents and Conditions
Hydrolysis: Typically carried out using aqueous acid or base.
Deprotection: Achieved using trifluoroacetic acid (TFA) in dichloromethane.
Major Products
Hydrolysis: Produces valine-alanine dipeptide and methanol.
Deprotection: Yields valine-alanine dipeptide with a free amine group.
Coupling: Forms longer peptide chains or cyclic peptides.
Scientific Research Applications
BOC-Val-Ala-OMe has a wide range of applications in scientific research:
Chemistry: Used as a building block in peptide synthesis and for studying peptide bond formation.
Biology: Serves as a model compound for studying protein folding and interactions.
Medicine: Investigated for its potential in drug delivery systems and as a precursor for bioactive peptides.
Industry: Utilized in the production of peptide-based materials and nanostructures.
Comparison with Similar Compounds
Similar Compounds
BOC-Val-Val-OMe: Another dipeptide derivative with similar protective groups and applications.
BOC-Ile-Ala-OMe: Contains isoleucine instead of valine, used in similar research contexts.
H-Lys-Ala-Val-Gly-OH: A lysine-containing tetrapeptide with immunostimulatory activity.
Uniqueness
BOC-Val-Ala-OMe is unique due to its specific combination of valine and alanine, which provides distinct properties in terms of stability and reactivity. Its ease of synthesis and versatility in forming peptide bonds make it a preferred choice in peptide research and industrial applications .
Properties
IUPAC Name |
methyl 2-[[3-methyl-2-[(2-methylpropan-2-yl)oxycarbonylamino]butanoyl]amino]propanoate | |
---|---|---|
Details | Computed by LexiChem 2.6.6 (PubChem release 2019.06.18) | |
Source | PubChem | |
URL | https://pubchem.ncbi.nlm.nih.gov | |
Description | Data deposited in or computed by PubChem | |
InChI |
InChI=1S/C14H26N2O5/c1-8(2)10(16-13(19)21-14(4,5)6)11(17)15-9(3)12(18)20-7/h8-10H,1-7H3,(H,15,17)(H,16,19) | |
Details | Computed by InChI 1.0.5 (PubChem release 2019.06.18) | |
Source | PubChem | |
URL | https://pubchem.ncbi.nlm.nih.gov | |
Description | Data deposited in or computed by PubChem | |
InChI Key |
GNOSSFVOMTYCFF-UHFFFAOYSA-N | |
Details | Computed by InChI 1.0.5 (PubChem release 2019.06.18) | |
Source | PubChem | |
URL | https://pubchem.ncbi.nlm.nih.gov | |
Description | Data deposited in or computed by PubChem | |
Canonical SMILES |
CC(C)C(C(=O)NC(C)C(=O)OC)NC(=O)OC(C)(C)C | |
Details | Computed by OEChem 2.1.5 (PubChem release 2019.06.18) | |
Source | PubChem | |
URL | https://pubchem.ncbi.nlm.nih.gov | |
Description | Data deposited in or computed by PubChem | |
Molecular Formula |
C14H26N2O5 | |
Details | Computed by PubChem 2.1 (PubChem release 2019.06.18) | |
Source | PubChem | |
URL | https://pubchem.ncbi.nlm.nih.gov | |
Description | Data deposited in or computed by PubChem | |
Molecular Weight |
302.37 g/mol | |
Details | Computed by PubChem 2.1 (PubChem release 2021.05.07) | |
Source | PubChem | |
URL | https://pubchem.ncbi.nlm.nih.gov | |
Description | Data deposited in or computed by PubChem | |
Retrosynthesis Analysis
AI-Powered Synthesis Planning: Our tool employs the Template_relevance Pistachio, Template_relevance Bkms_metabolic, Template_relevance Pistachio_ringbreaker, Template_relevance Reaxys, Template_relevance Reaxys_biocatalysis model, leveraging a vast database of chemical reactions to predict feasible synthetic routes.
One-Step Synthesis Focus: Specifically designed for one-step synthesis, it provides concise and direct routes for your target compounds, streamlining the synthesis process.
Accurate Predictions: Utilizing the extensive PISTACHIO, BKMS_METABOLIC, PISTACHIO_RINGBREAKER, REAXYS, REAXYS_BIOCATALYSIS database, our tool offers high-accuracy predictions, reflecting the latest in chemical research and data.
Strategy Settings
Precursor scoring | Relevance Heuristic |
---|---|
Min. plausibility | 0.01 |
Model | Template_relevance |
Template Set | Pistachio/Bkms_metabolic/Pistachio_ringbreaker/Reaxys/Reaxys_biocatalysis |
Top-N result to add to graph | 6 |
Feasible Synthetic Routes
Disclaimer and Information on In-Vitro Research Products
Please be aware that all articles and product information presented on BenchChem are intended solely for informational purposes. The products available for purchase on BenchChem are specifically designed for in-vitro studies, which are conducted outside of living organisms. In-vitro studies, derived from the Latin term "in glass," involve experiments performed in controlled laboratory settings using cells or tissues. It is important to note that these products are not categorized as medicines or drugs, and they have not received approval from the FDA for the prevention, treatment, or cure of any medical condition, ailment, or disease. We must emphasize that any form of bodily introduction of these products into humans or animals is strictly prohibited by law. It is essential to adhere to these guidelines to ensure compliance with legal and ethical standards in research and experimentation.