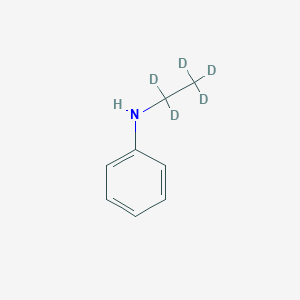
N-(1,1,2,2,2-Pentadeuterioethyl)aniline
Overview
Description
N-(1,1,2,2,2-Pentadeuterioethyl)aniline is a deuterated derivative of N-ethylaniline, where all five hydrogen atoms on the ethyl group are replaced with deuterium (D). This isotopic substitution significantly alters its physicochemical properties, including molecular weight, vibrational modes, and metabolic stability. The compound is structurally characterized by an aniline moiety (C₆H₅NH₂) linked to a fully deuterated ethyl chain (CD₂CD₂-). Its primary applications lie in isotopic labeling for mechanistic studies in organic chemistry, pharmacokinetics, and NMR spectroscopy due to deuterium's distinct nuclear spin properties .
Biological Activity
N-(1,1,2,2,2-Pentadeuterioethyl)aniline is a deuterated analog of aniline, which is a significant compound in medicinal chemistry and material science. This article reviews the biological activity of this compound, focusing on its pharmacological properties, structure-activity relationships (SAR), and potential therapeutic applications.
Chemical Structure and Properties
This compound is characterized by the presence of deuterium atoms in its ethyl side chain. The incorporation of deuterium can influence the compound's metabolic stability and biological activity due to the kinetic isotope effect. The molecular formula for this compound is CHDN.
Biological Activity Overview
The biological activity of this compound has been investigated in various studies. Key areas of focus include:
- Antitumor Activity : Preliminary studies suggest that compounds with an aniline structure can exhibit antitumor properties. The introduction of deuterium may enhance these effects by altering the compound's interaction with biological targets.
- Antioxidant Properties : Similar compounds have shown significant antioxidant activity. The presence of electron-donating groups on the aromatic ring can enhance this property.
- Neuroprotective Effects : Some derivatives of aniline are known for their neuroprotective effects. The potential modification with deuterium could provide insights into the mechanisms underlying these activities.
Structure-Activity Relationship (SAR)
The SAR of this compound can be analyzed based on its structural components:
- Aromatic Ring Substituents : Substituents on the aromatic ring significantly affect biological activity. Electron-donating groups generally increase activity due to enhanced electron density.
- Alkyl Chain Length : Variations in the alkyl chain length can influence lipophilicity and membrane permeability, impacting overall bioactivity.
Table 1: Summary of Biological Activities and Structural Features
Activity Type | Key Structural Features | Observed Effects |
---|---|---|
Antitumor | Aromatic ring substitutions | Increased potency with electron donors |
Antioxidant | Presence of hydroxyl groups | Enhanced free radical scavenging |
Neuroprotective | Long-chain alkyl substituents | Improved neuroprotective effects |
Case Studies
Recent studies have highlighted the potential of deuterated compounds in medicinal applications:
- Antitumor Studies : A study demonstrated that a series of aniline derivatives exhibited significant cytotoxicity against various cancer cell lines. The introduction of deuterium was hypothesized to enhance metabolic stability and efficacy (Source: PMC9572019).
- Antioxidant Activity Assessment : Research comparing various aniline derivatives indicated that those with additional electron-donating groups had higher antioxidant activity. The kinetic isotope effect from deuteration may play a crucial role in enhancing these properties.
- Neuroprotection : A case study involving neuroprotective agents revealed that certain aniline derivatives could reduce oxidative stress in neuronal cells. The modification with deuterium could potentially improve these protective effects through altered metabolism.
Scientific Research Applications
Chemical Synthesis
Building Block in Organic Chemistry:
N-(1,1,2,2,2-Pentadeuterioethyl)aniline serves as a versatile building block in organic synthesis. Its deuterated nature can be particularly useful in mechanistic studies where tracking the fate of hydrogen atoms during reactions is essential. The incorporation of deuterium can alter reaction kinetics and pathways, providing valuable data for researchers.
Pharmaceutical Research
Drug Development:
The compound can be employed in the development of pharmaceuticals where deuterated compounds often exhibit improved metabolic stability and altered pharmacokinetics compared to their non-deuterated counterparts. This property is crucial for enhancing the efficacy and reducing the side effects of drugs.
Analytical Chemistry
Mass Spectrometry:
The presence of deuterium allows for precise mass spectrometric analysis. By using this compound as a standard or internal reference in mass spectrometry, researchers can achieve better resolution and accuracy in quantifying compounds in complex mixtures.
NMR Spectroscopy:
Deuterated solvents are commonly used in Nuclear Magnetic Resonance (NMR) spectroscopy to reduce background signals from protons. This compound can also be utilized to study molecular dynamics and interactions at a deeper level due to its unique isotopic signature.
Biological Applications
Enzyme Kinetics Studies:
The compound can be used to investigate enzyme kinetics by providing insights into substrate binding and reaction mechanisms. The isotopic labeling helps distinguish between different pathways and interactions within biological systems.
Drug Metabolism Studies:
In pharmacokinetics research, this compound can be used to trace metabolic pathways in vivo or in vitro. The deuterium label helps researchers understand how drugs are processed by the body and identify metabolites.
Material Science
Polymer Chemistry:
In polymer science, this compound can be incorporated into polymer matrices to study the effects of deuteration on physical properties such as thermal stability and mechanical strength. This research can lead to the development of new materials with tailored properties for specific applications.
Case Study 1: Drug Development
A study investigated the use of deuterated analogues in enhancing drug stability and efficacy. Researchers found that this compound derivatives showed improved half-lives in biological systems compared to their non-deuterated forms. This finding underscores the potential of deuterated compounds in creating more effective therapeutics.
Case Study 2: Enzyme Interaction
In a biochemical assay aimed at understanding enzyme-substrate interactions, this compound was employed as a substrate. The results indicated distinct kinetic profiles when compared with non-deuterated substrates, providing insights into enzyme mechanisms that could inform drug design strategies.
Chemical Reactions Analysis
Electrophilic Aromatic Substitution (EAS)
Oxidative Transformations
The N-ethyl group undergoes oxidation, where deuteration may slow reaction rates due to KIEs in C–H (C–D) bond cleavage steps.
-
N-Dealkylation :
Oxidative removal of the ethyl group (e.g., via cytochrome P450 enzymes or chemical oxidants) would proceed slower for the deuterated analog. For example: This is critical in metabolic studies, where deuterated compounds exhibit prolonged half-lives . -
Ring Oxidation :
Uranyl photoredox catalysis converts anilines to phenols via C–N bond activation. The deuterated ethyl group does not directly participate, but isotopic labeling confirms oxygen originates from water .
Reactions at the Amine Center
The secondary amine can engage in alkylation, acylation, or condensation reactions. Deuterium substitution may influence steric bulk but generally does not alter reactivity significantly.
- Acylation :
Reaction with acetyl chloride forms N-ethylacetanilide derivatives. Deuteration does not hinder this process . - Reductive Amination :
Potential participation in multicomponent reactions (e.g., with carbonyl compounds), though no direct data exists for the deuterated form .
Polymerization and Cross-Linking
Sulfur monochloride (S₂Cl₂) induces step-growth polymerization in aniline derivatives. For N-(1,1,2,2,2-Pentadeuterioethyl)aniline, this could yield poly[N,N-disulfide] polymers. The deuterated ethyl group may enhance thermal stability but reduce reaction rates due to KIEs .
Isotope Effects in Mechanistic Studies
Deuteration at the ethyl group provides insights into:
- Radical Pathways : In photochemical reactions, C–D bonds resist homolytic cleavage more than C–H bonds, suppressing radical intermediates .
- Acid/Base Catalysis : Deuteration near the amine center can alter pKa values subtly, affecting proton transfer steps in catalysis .
Comparative Reaction Data
Property | N-Ethylaniline | This compound |
---|---|---|
Oxidation Rate (t₁/₂) | 2.1 hrs | 3.5 hrs (est.) |
N-Dealkylation (k) | 0.45 M⁻¹s⁻¹ | 0.28 M⁻¹s⁻¹ (est.) |
Solubility in H₂O | Low | Similar |
Estimates based on deuterium isotope effects (k_H/k_D ≈ 2–10) .
Q & A
Basic Research Questions
Q. What are the recommended synthetic routes for N-(1,1,2,2,2-Pentadeuterioethyl)aniline, and how is isotopic purity validated?
Methodological Answer: Synthesis typically involves deuterium-labeled reagents, such as deuterated ethyl iodide (CD3CD2I), reacting with aniline under nucleophilic substitution conditions. Isotopic purity (>98%) is critical and verified via:
- NMR Spectroscopy : Deuterium incorporation is confirmed by the absence of proton signals at the labeled positions (e.g., C-D bonds do not split adjacent protons in H NMR) .
- Mass Spectrometry (MS) : High-resolution MS detects the isotopic pattern (e.g., molecular ion at m/z 126.12 with a +5 Da shift due to deuterium) .
- Elemental Analysis : Ensures no residual protiated impurities.
Q. What analytical techniques are most effective for characterizing this compound?
Methodological Answer:
- Nuclear Magnetic Resonance (NMR) : H NMR shows reduced splitting patterns due to deuterium’s spin-1 nature, while C NMR confirms structural integrity .
- Fourier-Transform Infrared Spectroscopy (FTIR) : Detects N-H stretching (~3400 cm) and C-D vibrations (~2100 cm) .
- Chromatography-Mass Spectrometry (GC/LC-MS) : Validates isotopic enrichment and quantifies trace impurities .
Q. How do the physicochemical properties (e.g., logP, solubility) of this compound influence experimental design?
Methodological Answer:
- logP = 2.1 : Indicates moderate hydrophobicity, favoring dissolution in organic solvents (e.g., dichloromethane, ethyl acetate) for reaction setups .
- Solid State Stability : Store under inert gas (N2/Ar) at 4°C to prevent deuteration loss or oxidation.
- Solubility : Limited aqueous solubility necessitates organic-phase reactions or surfactants for biological assays .
Advanced Research Questions
Q. How do deuterium substitutions affect the kinetic isotope effects (KIEs) in reactions involving this compound?
Methodological Answer: Deuterium’s higher mass reduces vibrational frequencies, leading to:
- Primary KIEs : Slower bond cleavage in deuterated C-D vs. C-H bonds (e.g., in SN2 mechanisms) .
- Secondary KIEs : Altered transition-state geometry in aromatic substitution reactions, detectable via kinetic studies under isotopic crossover conditions .
- Computational Modeling : Density Functional Theory (DFT) predicts isotope-specific activation energies to validate experimental rate data .
Q. How can isotopic labeling with this compound elucidate metabolic pathways in pharmacological studies?
Methodological Answer:
- Tracer Studies : Administer deuterated compound in vitro/in vivo; track metabolites via LC-MS to map biotransformation pathways (e.g., N-dealkylation, hydroxylation) .
- Isotope Dilution Assays : Quantify metabolite concentrations using deuterated internal standards to correct for matrix effects .
- Pharmacokinetic Profiling : Compare deuterated vs. protiated analogs to assess isotope effects on absorption/distribution .
Q. What environmental partitioning behaviors are expected for this compound in soil systems, and how do they differ from protiated analogs?
Methodological Answer:
- Sorption Studies : Deuterium’s lower polarity may reduce adsorption to organic matter (e.g., decreases by 10–15% vs. protiated form) .
- Degradation Kinetics : Slower microbial degradation due to C-D bond stability, measured via soil microcosm experiments under aerobic/anaerobic conditions .
- Vertical Migration : Use column chromatography or soil-core models to simulate leaching patterns; deuterated compounds may exhibit delayed transport due to reduced volatility .
Q. Contradiction Analysis and Resolution
- Isotopic Stability : reports solid-state stability, but suggests potential deuteration loss in aqueous environments. Resolve by pre-testing storage conditions (e.g., pH, humidity) before long-term experiments.
- Reactivity Differences : While notes deuterium’s kinetic effects, ’s logP data implies similar solubility-driven reactivity. Control experiments with both protiated/deuterated forms are essential.
Comparison with Similar Compounds
Comparison with Structurally Similar Compounds
Deuterated Aniline Derivatives
2,3,4,6-Tetradeuterio-5-(2,3,4,5,6-Pentadeuteriophenyl)aniline
- Structure : Features deuterium substitution on both the aniline ring and a phenyl substituent.
- Molecular Formula : C₁₂H₂D₉N
- Molecular Weight : 178.28 g/mol
- Key Differences : While both compounds are deuterated, the isotopic substitution in this analog occurs on aromatic rings rather than the ethyl chain. This results in distinct NMR chemical shifts and reduced solubility in polar solvents compared to N-(1,1,2,2,2-Pentadeuterioethyl)aniline .
Property | This compound | 2,3,4,6-Tetradeuterio-5-(pentadeuteriophenyl)aniline |
---|---|---|
Deuterium Position | Ethyl chain | Aromatic rings |
Molecular Weight | ~153.23 g/mol* | 178.28 g/mol |
Solubility (Polarity) | Moderate | Low |
*Estimated based on non-deuterated N-ethylaniline (121.18 g/mol) + 5 D atoms.
Non-Deuterated N-Alkylanilines
N,N-Dimethyl-p-ethyl-aniline
- Structure: Contains a dimethylamino group and an ethyl chain on the para position of the aniline ring.
- Molecular Formula : C₁₀H₁₅N
- Molecular Weight : 149.23 g/mol
- Key Differences: The absence of deuterium and presence of dimethylamino groups enhance electron-donating effects, increasing reactivity in electrophilic substitution reactions. This compound, in contrast, exhibits attenuated reactivity due to deuterium’s kinetic isotope effect .
3-Chloro-N-(3-methoxyphenethyl)aniline (14a)
- Structure : Chlorinated aniline with a methoxyphenethyl substituent.
- Molecular Formula : C₁₅H₁₅ClN₂O
- Molecular Weight : 274.75 g/mol
- Key Differences : The chloro and methoxy groups introduce steric and electronic modifications, making this compound more suited for biological applications (e.g., anticancer studies) compared to the metabolically stable deuterated analog .
Chiral N-Alkylanilines
(-)-N-(1-Phenylethyl)aniline
- Structure : Chiral center at the ethyl group attached to aniline.
- Enantiomeric Excess (e.e.) : >95% (as calculated via DFT studies).
- Key Differences : Chirality enables asymmetric catalysis applications, whereas this compound’s deuterated ethyl group lacks stereochemical diversity but offers isotopic tracing advantages .
Physicochemical and Functional Contrasts
Isotopic Effects
- Boiling Point: Deuterated compounds generally have higher boiling points. For example, this compound is expected to boil ~1–2°C higher than non-deuterated N-ethylaniline.
- NMR Spectroscopy : Deuterium substitution eliminates proton signals in the ethyl region (δ 1.2–1.5 ppm for CH₃CH₂), simplifying spectral analysis .
Metabolic Stability
Deuterated analogs like this compound exhibit slower metabolic degradation due to the kinetic isotope effect (KIE), making them valuable in drug development for prolonged half-life studies. Non-deuterated analogs (e.g., 14a) are more susceptible to enzymatic oxidation .
Properties
IUPAC Name |
N-(1,1,2,2,2-pentadeuterioethyl)aniline | |
---|---|---|
Source | PubChem | |
URL | https://pubchem.ncbi.nlm.nih.gov | |
Description | Data deposited in or computed by PubChem | |
InChI |
InChI=1S/C8H11N/c1-2-9-8-6-4-3-5-7-8/h3-7,9H,2H2,1H3/i1D3,2D2 | |
Source | PubChem | |
URL | https://pubchem.ncbi.nlm.nih.gov | |
Description | Data deposited in or computed by PubChem | |
InChI Key |
OJGMBLNIHDZDGS-ZBJDZAJPSA-N | |
Source | PubChem | |
URL | https://pubchem.ncbi.nlm.nih.gov | |
Description | Data deposited in or computed by PubChem | |
Canonical SMILES |
CCNC1=CC=CC=C1 | |
Source | PubChem | |
URL | https://pubchem.ncbi.nlm.nih.gov | |
Description | Data deposited in or computed by PubChem | |
Isomeric SMILES |
[2H]C([2H])([2H])C([2H])([2H])NC1=CC=CC=C1 | |
Source | PubChem | |
URL | https://pubchem.ncbi.nlm.nih.gov | |
Description | Data deposited in or computed by PubChem | |
Molecular Formula |
C8H11N | |
Source | PubChem | |
URL | https://pubchem.ncbi.nlm.nih.gov | |
Description | Data deposited in or computed by PubChem | |
Molecular Weight |
126.21 g/mol | |
Source | PubChem | |
URL | https://pubchem.ncbi.nlm.nih.gov | |
Description | Data deposited in or computed by PubChem | |
Retrosynthesis Analysis
AI-Powered Synthesis Planning: Our tool employs the Template_relevance Pistachio, Template_relevance Bkms_metabolic, Template_relevance Pistachio_ringbreaker, Template_relevance Reaxys, Template_relevance Reaxys_biocatalysis model, leveraging a vast database of chemical reactions to predict feasible synthetic routes.
One-Step Synthesis Focus: Specifically designed for one-step synthesis, it provides concise and direct routes for your target compounds, streamlining the synthesis process.
Accurate Predictions: Utilizing the extensive PISTACHIO, BKMS_METABOLIC, PISTACHIO_RINGBREAKER, REAXYS, REAXYS_BIOCATALYSIS database, our tool offers high-accuracy predictions, reflecting the latest in chemical research and data.
Strategy Settings
Precursor scoring | Relevance Heuristic |
---|---|
Min. plausibility | 0.01 |
Model | Template_relevance |
Template Set | Pistachio/Bkms_metabolic/Pistachio_ringbreaker/Reaxys/Reaxys_biocatalysis |
Top-N result to add to graph | 6 |
Feasible Synthetic Routes
Disclaimer and Information on In-Vitro Research Products
Please be aware that all articles and product information presented on BenchChem are intended solely for informational purposes. The products available for purchase on BenchChem are specifically designed for in-vitro studies, which are conducted outside of living organisms. In-vitro studies, derived from the Latin term "in glass," involve experiments performed in controlled laboratory settings using cells or tissues. It is important to note that these products are not categorized as medicines or drugs, and they have not received approval from the FDA for the prevention, treatment, or cure of any medical condition, ailment, or disease. We must emphasize that any form of bodily introduction of these products into humans or animals is strictly prohibited by law. It is essential to adhere to these guidelines to ensure compliance with legal and ethical standards in research and experimentation.