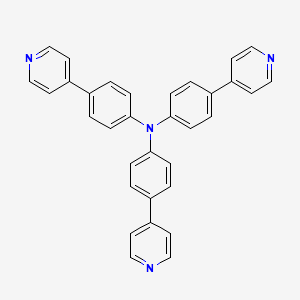
Tris(4-(pyridin-4-yl)phenyl)amine
Overview
Description
Tris(4-(pyridin-4-yl)phenyl)amine is a useful research compound. Its molecular formula is C33H24N4 and its molecular weight is 476.6 g/mol. The purity is usually 95%.
BenchChem offers high-quality this compound suitable for many research applications. Different packaging options are available to accommodate customers' requirements. Please inquire for more information about this compound including the price, delivery time, and more detailed information at info@benchchem.com.
Mechanism of Action
Target of Action
Tris(4-(pyridin-4-yl)phenyl)amine, also known as NPy3, is primarily used as a redox-active ligand in coordination frameworks . It has been incorporated into various frameworks such as those involving Mn(II) and Cu(I) . These frameworks serve as the primary targets of NPy3.
Mode of Action
NPy3 interacts with its targets by facilitating redox state switching in the materials . This is achieved through the monoradical cation of the ligand, which is readily accessible and enables chemical oxidation of the framework . The redox properties of the framework are investigated, and the oxidised state of the framework is generated chemically .
Biochemical Pathways
The compound’s ability to facilitate redox state switching suggests it may influence electron transfer processes within the coordination frameworks .
Result of Action
The primary result of NPy3’s action is the facilitation of redox state switching in the materials of the coordination frameworks . This can lead to changes in the frameworks’ electrochemical and spectral properties . For instance, a Mn(II) coordination framework incorporating NPy3 was found to exhibit multifunctionality by virtue of its ability to switch the fluorescence ‘on’ and ‘off’ with the redox state .
Action Environment
The action of NPy3 can be influenced by various environmental factors. For instance, the initial metal centre in the coordination frameworks was reduced in situ during the solvothermal synthesis under relatively mild conditions . Additionally, the compound may be sensitive to light and moisture, and it is typically stored under dry conditions and away from light to maintain its stability .
Preparation Methods
Industrial Production Methods
Chemical Reactions Analysis
Types of Reactions
Tris(4-(pyridin-4-yl)phenyl)amine undergoes various chemical reactions, including:
Oxidation: The compound can be oxidized to form radical cations, which are useful in redox-active materials.
Common Reagents and Conditions
Oxidation: Common oxidizing agents include ferric chloride and ceric ammonium nitrate.
Reduction: Reducing agents such as sodium borohydride and lithium aluminum hydride are used.
Substitution: Reagents like alkyl halides and acyl chlorides are employed in substitution reactions.
Major Products
The major products formed from these reactions depend on the specific reagents and conditions used. For example, oxidation can yield radical cations, while substitution reactions can introduce various functional groups onto the pyridinyl rings .
Scientific Research Applications
Tris(4-(pyridin-4-yl)phenyl)amine has a wide range of applications in scientific research:
Industry: It is employed in the development of organic light-emitting diodes (OLEDs) and other optoelectronic devices due to its excellent electron transport properties[][1].
Comparison with Similar Compounds
Similar Compounds
Triphenylamine: Similar in structure but lacks the pyridinyl groups, resulting in different electronic properties.
Tris(4-(pyridin-3-yl)phenyl)amine: Similar but with pyridinyl groups in the 3-position, affecting its reactivity and coordination behavior.
Uniqueness
Tris(4-(pyridin-4-yl)phenyl)amine is unique due to the presence of pyridinyl groups in the 4-position, which enhances its ability to participate in coordination chemistry and redox reactions. This makes it particularly valuable in the development of advanced materials for electronic and optoelectronic applications .
Properties
IUPAC Name |
4-pyridin-4-yl-N,N-bis(4-pyridin-4-ylphenyl)aniline | |
---|---|---|
Details | Computed by Lexichem TK 2.7.0 (PubChem release 2021.05.07) | |
Source | PubChem | |
URL | https://pubchem.ncbi.nlm.nih.gov | |
Description | Data deposited in or computed by PubChem | |
InChI |
InChI=1S/C33H24N4/c1-7-31(8-2-25(1)28-13-19-34-20-14-28)37(32-9-3-26(4-10-32)29-15-21-35-22-16-29)33-11-5-27(6-12-33)30-17-23-36-24-18-30/h1-24H | |
Details | Computed by InChI 1.0.6 (PubChem release 2021.05.07) | |
Source | PubChem | |
URL | https://pubchem.ncbi.nlm.nih.gov | |
Description | Data deposited in or computed by PubChem | |
InChI Key |
CRFFXCBSBSCEPT-UHFFFAOYSA-N | |
Details | Computed by InChI 1.0.6 (PubChem release 2021.05.07) | |
Source | PubChem | |
URL | https://pubchem.ncbi.nlm.nih.gov | |
Description | Data deposited in or computed by PubChem | |
Canonical SMILES |
C1=CC(=CC=C1C2=CC=NC=C2)N(C3=CC=C(C=C3)C4=CC=NC=C4)C5=CC=C(C=C5)C6=CC=NC=C6 | |
Details | Computed by OEChem 2.3.0 (PubChem release 2021.05.07) | |
Source | PubChem | |
URL | https://pubchem.ncbi.nlm.nih.gov | |
Description | Data deposited in or computed by PubChem | |
Molecular Formula |
C33H24N4 | |
Details | Computed by PubChem 2.1 (PubChem release 2021.05.07) | |
Source | PubChem | |
URL | https://pubchem.ncbi.nlm.nih.gov | |
Description | Data deposited in or computed by PubChem | |
Molecular Weight |
476.6 g/mol | |
Details | Computed by PubChem 2.1 (PubChem release 2021.05.07) | |
Source | PubChem | |
URL | https://pubchem.ncbi.nlm.nih.gov | |
Description | Data deposited in or computed by PubChem | |
Retrosynthesis Analysis
AI-Powered Synthesis Planning: Our tool employs the Template_relevance Pistachio, Template_relevance Bkms_metabolic, Template_relevance Pistachio_ringbreaker, Template_relevance Reaxys, Template_relevance Reaxys_biocatalysis model, leveraging a vast database of chemical reactions to predict feasible synthetic routes.
One-Step Synthesis Focus: Specifically designed for one-step synthesis, it provides concise and direct routes for your target compounds, streamlining the synthesis process.
Accurate Predictions: Utilizing the extensive PISTACHIO, BKMS_METABOLIC, PISTACHIO_RINGBREAKER, REAXYS, REAXYS_BIOCATALYSIS database, our tool offers high-accuracy predictions, reflecting the latest in chemical research and data.
Strategy Settings
Precursor scoring | Relevance Heuristic |
---|---|
Min. plausibility | 0.01 |
Model | Template_relevance |
Template Set | Pistachio/Bkms_metabolic/Pistachio_ringbreaker/Reaxys/Reaxys_biocatalysis |
Top-N result to add to graph | 6 |
Feasible Synthetic Routes
Q1: What are the structural characteristics of Tris(4-(pyridin-4-yl)phenyl)amine?
A1: this compound (NPy3) is an organic compound with the molecular formula C33H24N4 []. Its structure consists of a central nitrogen atom connected to three phenyl rings, each substituted with a pyridin-4-yl group at the para position. This tripodal arrangement of pyridine rings makes it a versatile ligand for coordination chemistry.
Q2: What makes this compound interesting for material science applications?
A2: NPy3 stands out due to its redox activity. The triarylamine core can undergo a one-electron oxidation to form a stable radical cation []. This property makes it attractive for developing multifunctional materials, particularly in the realm of electroactive Metal-Organic Frameworks (MOFs).
Q3: How does this compound behave within Metal-Organic Frameworks?
A3: NPy3 acts as a bridging ligand, coordinating metal ions through its nitrogen atoms to form extended framework structures [, ]. The choice of metal ion and co-ligands allows for tuning the framework's properties, including its redox behavior.
Q4: Can you provide examples of how this compound-based MOFs have been utilized?
A4: Researchers have successfully incorporated NPy3 into frameworks with various metal ions like Cu(II) [], Mn(II) [], and Co(II) [, , ]. These materials exhibit interesting electrochemical and optical properties, with potential applications in areas like sensing and electrochromic devices. For example, a Mn(II) framework containing NPy3 showed the ability to switch its fluorescence "on" and "off" depending on its redox state [].
Q5: How does the steric bulk of co-ligands influence the structure of this compound-based MOFs?
A5: Studies have shown that using co-ligands with increasing steric bulk can affect the degree of interpenetration in the resulting MOFs. For instance, using bulkier dicarboxylate co-ligands like 2,3,5,6-tetramethyl-1,4-benzenedicarboxylate led to non-interpenetrated structures, while less hindered options resulted in 3-fold interpenetrated networks [].
Q6: Has this compound been explored for biological applications?
A6: Yes, NPy3 has shown potential in bioimaging applications. A macromolecule incorporating NPy3 demonstrated specific nucleolus-targeting ability and aggregation-induced emission (AIE) properties, making it a promising candidate for live-cell imaging [].
Q7: Are there any studies on the environmental impact or degradation of this compound?
A7: Currently, the provided research papers do not offer specific information regarding the environmental impact, degradation pathways, or potential ecotoxicological effects of NPy3. Further investigation is needed to understand its fate and behavior in the environment.
Q8: What analytical techniques are commonly employed to study this compound and its derivatives?
A8: Researchers utilize a variety of techniques to characterize NPy3 and its derivatives, including:
- Spectroscopic methods: NMR (1H and 13C) [], IR [, ], UV-Vis [, , ], fluorescence spectroscopy [, , ], and EPR [] are used to analyze structure, electronic properties, and redox behavior.
- Electrochemical methods: Cyclic voltammetry and spectroelectrochemistry provide insights into redox potentials and stability of different oxidation states [, , , , ].
- X-ray diffraction: Single-crystal X-ray diffraction helps determine the solid-state structures of NPy3-containing compounds and MOFs [, ].
Disclaimer and Information on In-Vitro Research Products
Please be aware that all articles and product information presented on BenchChem are intended solely for informational purposes. The products available for purchase on BenchChem are specifically designed for in-vitro studies, which are conducted outside of living organisms. In-vitro studies, derived from the Latin term "in glass," involve experiments performed in controlled laboratory settings using cells or tissues. It is important to note that these products are not categorized as medicines or drugs, and they have not received approval from the FDA for the prevention, treatment, or cure of any medical condition, ailment, or disease. We must emphasize that any form of bodily introduction of these products into humans or animals is strictly prohibited by law. It is essential to adhere to these guidelines to ensure compliance with legal and ethical standards in research and experimentation.