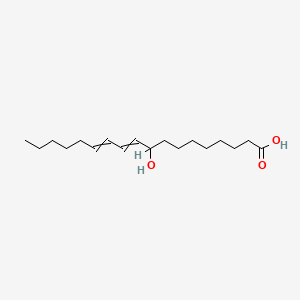
9-Hydroxyoctadeca-10,12-dienoic acid
Overview
Description
9-Hydroxyoctadeca-10,12-dienoic acid is a hydroxylated derivative of linoleic acid, an essential fatty acid. It is known for its role as a metabolite in various biological processes and has been studied for its potential implications in health and disease .
Preparation Methods
Synthetic Routes and Reaction Conditions
9-Hydroxyoctadeca-10,12-dienoic acid can be synthesized through the hydroxylation of linoleic acid. This process typically involves the use of enzymes such as cyclooxygenase 1 (COX-1) and cyclooxygenase 2 (COX-2), which convert linoleic acid to 9-hydroperoxy-10,12-octadecadienoic acid. This intermediate is then reduced to form this compound .
Industrial Production Methods
Industrial production methods for this compound are not well-documented. the enzymatic conversion of linoleic acid using bioreactors could be a potential method for large-scale production.
Chemical Reactions Analysis
Types of Reactions
9-Hydroxyoctadeca-10,12-dienoic acid undergoes various chemical reactions, including:
Oxidation: It can be further oxidized to form 9-oxo-10,12-octadecadienoic acid.
Reduction: The hydroperoxy intermediate can be reduced to form the hydroxylated product.
Substitution: The hydroxyl group can participate in substitution reactions under appropriate conditions.
Common Reagents and Conditions
Oxidation: Common oxidizing agents include hydrogen peroxide and molecular oxygen.
Reduction: Reducing agents such as sodium borohydride or enzymatic reduction can be used.
Substitution: Various nucleophiles can be used for substitution reactions, depending on the desired product.
Major Products
Oxidation: 9-oxo-10,12-octadecadienoic acid.
Reduction: this compound.
Substitution: Various substituted derivatives depending on the nucleophile used.
Scientific Research Applications
9-Hydroxyoctadeca-10,12-dienoic acid has several scientific research applications:
Chemistry: It is used as a model compound to study the oxidation and reduction of fatty acids.
Biology: It plays a role in cellular signaling and has been implicated in inflammatory responses.
Medicine: It is studied for its potential role in diseases such as cancer and cardiovascular diseases.
Industry: It can be used in the production of bio-based materials and as a precursor for various chemical syntheses
Mechanism of Action
9-Hydroxyoctadeca-10,12-dienoic acid exerts its effects by binding to peroxisome proliferator-activated receptors (PPARs), particularly PPAR gamma. This binding modulates the transcription of target genes involved in fatty acid metabolism, inflammation, and glucose homeostasis. The compound acts as a key regulator of adipocyte differentiation and glucose homeostasis .
Comparison with Similar Compounds
Similar Compounds
13-Hydroxyoctadeca-9,11-dienoic acid: Another hydroxylated derivative of linoleic acid with similar biological activities.
9-oxo-10,12-octadecadienoic acid: An oxidized form of 9-Hydroxyoctadeca-10,12-dienoic acid with distinct biological properties.
13-oxo-9,11-octadecadienoic acid: An oxidized derivative of 13-Hydroxyoctadeca-9,11-dienoic acid
Uniqueness
This compound is unique due to its specific hydroxylation pattern and its ability to modulate PPAR gamma activity. This makes it a valuable compound for studying fatty acid metabolism and its implications in health and disease .
Properties
IUPAC Name |
9-hydroxyoctadeca-10,12-dienoic acid | |
---|---|---|
Details | Computed by Lexichem TK 2.7.0 (PubChem release 2021.05.07) | |
Source | PubChem | |
URL | https://pubchem.ncbi.nlm.nih.gov | |
Description | Data deposited in or computed by PubChem | |
InChI |
InChI=1S/C18H32O3/c1-2-3-4-5-6-8-11-14-17(19)15-12-9-7-10-13-16-18(20)21/h6,8,11,14,17,19H,2-5,7,9-10,12-13,15-16H2,1H3,(H,20,21) | |
Details | Computed by InChI 1.0.6 (PubChem release 2021.05.07) | |
Source | PubChem | |
URL | https://pubchem.ncbi.nlm.nih.gov | |
Description | Data deposited in or computed by PubChem | |
InChI Key |
NPDSHTNEKLQQIJ-UHFFFAOYSA-N | |
Details | Computed by InChI 1.0.6 (PubChem release 2021.05.07) | |
Source | PubChem | |
URL | https://pubchem.ncbi.nlm.nih.gov | |
Description | Data deposited in or computed by PubChem | |
Canonical SMILES |
CCCCCC=CC=CC(CCCCCCCC(=O)O)O | |
Details | Computed by OEChem 2.3.0 (PubChem release 2021.05.07) | |
Source | PubChem | |
URL | https://pubchem.ncbi.nlm.nih.gov | |
Description | Data deposited in or computed by PubChem | |
Molecular Formula |
C18H32O3 | |
Details | Computed by PubChem 2.1 (PubChem release 2021.05.07) | |
Source | PubChem | |
URL | https://pubchem.ncbi.nlm.nih.gov | |
Description | Data deposited in or computed by PubChem | |
DSSTOX Substance ID |
DTXSID20868260 | |
Record name | 9-Hydroxyoctadeca-10,12-dienoic acid | |
Source | EPA DSSTox | |
URL | https://comptox.epa.gov/dashboard/DTXSID20868260 | |
Description | DSSTox provides a high quality public chemistry resource for supporting improved predictive toxicology. | |
Molecular Weight |
296.4 g/mol | |
Details | Computed by PubChem 2.1 (PubChem release 2021.05.07) | |
Source | PubChem | |
URL | https://pubchem.ncbi.nlm.nih.gov | |
Description | Data deposited in or computed by PubChem | |
CAS No. |
15514-85-9 | |
Record name | 9-Hydroxy-10,12-octadecadienoic acid | |
Source | CAS Common Chemistry | |
URL | https://commonchemistry.cas.org/detail?cas_rn=15514-85-9 | |
Description | CAS Common Chemistry is an open community resource for accessing chemical information. Nearly 500,000 chemical substances from CAS REGISTRY cover areas of community interest, including common and frequently regulated chemicals, and those relevant to high school and undergraduate chemistry classes. This chemical information, curated by our expert scientists, is provided in alignment with our mission as a division of the American Chemical Society. | |
Explanation | The data from CAS Common Chemistry is provided under a CC-BY-NC 4.0 license, unless otherwise stated. | |
Record name | 9-Hydroxyoctadeca-10,12-dienoic acid | |
Source | EPA DSSTox | |
URL | https://comptox.epa.gov/dashboard/DTXSID20868260 | |
Description | DSSTox provides a high quality public chemistry resource for supporting improved predictive toxicology. | |
Retrosynthesis Analysis
AI-Powered Synthesis Planning: Our tool employs the Template_relevance Pistachio, Template_relevance Bkms_metabolic, Template_relevance Pistachio_ringbreaker, Template_relevance Reaxys, Template_relevance Reaxys_biocatalysis model, leveraging a vast database of chemical reactions to predict feasible synthetic routes.
One-Step Synthesis Focus: Specifically designed for one-step synthesis, it provides concise and direct routes for your target compounds, streamlining the synthesis process.
Accurate Predictions: Utilizing the extensive PISTACHIO, BKMS_METABOLIC, PISTACHIO_RINGBREAKER, REAXYS, REAXYS_BIOCATALYSIS database, our tool offers high-accuracy predictions, reflecting the latest in chemical research and data.
Strategy Settings
Precursor scoring | Relevance Heuristic |
---|---|
Min. plausibility | 0.01 |
Model | Template_relevance |
Template Set | Pistachio/Bkms_metabolic/Pistachio_ringbreaker/Reaxys/Reaxys_biocatalysis |
Top-N result to add to graph | 6 |
Feasible Synthetic Routes
Disclaimer and Information on In-Vitro Research Products
Please be aware that all articles and product information presented on BenchChem are intended solely for informational purposes. The products available for purchase on BenchChem are specifically designed for in-vitro studies, which are conducted outside of living organisms. In-vitro studies, derived from the Latin term "in glass," involve experiments performed in controlled laboratory settings using cells or tissues. It is important to note that these products are not categorized as medicines or drugs, and they have not received approval from the FDA for the prevention, treatment, or cure of any medical condition, ailment, or disease. We must emphasize that any form of bodily introduction of these products into humans or animals is strictly prohibited by law. It is essential to adhere to these guidelines to ensure compliance with legal and ethical standards in research and experimentation.