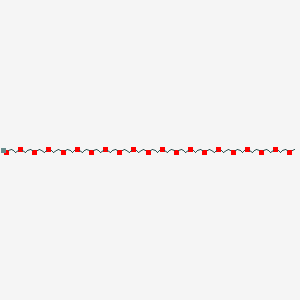
m-PEG20-alcohol
- Click on QUICK INQUIRY to receive a quote from our team of experts.
- With the quality product at a COMPETITIVE price, you can focus more on your research.
Overview
Description
Mechanism of Action
Target of Action
m-PEG20-alcohol is a PEG-based PROTAC linker . The primary targets of this compound are proteins that can be degraded by the ubiquitin-proteasome system . This system is crucial for maintaining cellular homeostasis by controlling the degradation of various proteins .
Mode of Action
This compound operates by linking two different ligands; one ligand is for an E3 ubiquitin ligase and the other is for the target protein . This forms a PROTAC molecule that can interact with its targets and induce changes. Specifically, PROTACs exploit the intracellular ubiquitin-proteasome system to selectively degrade target proteins .
Biochemical Pathways
The key biochemical pathway involved in the action of this compound is the ubiquitin-proteasome system . This system is responsible for protein degradation within cells, thereby controlling the concentration of specific proteins and maintaining cellular homeostasis .
Pharmacokinetics
It’s worth noting that the pharmacokinetics of a similar compound, uricase-peg 20, has been studied . Uricase-PEG 20 demonstrated a long half-life, suggesting less frequent dosing may be required
Result of Action
The primary result of this compound’s action is the selective degradation of target proteins . By exploiting the ubiquitin-proteasome system, this compound can control the concentration of specific proteins within cells . This can have various molecular and cellular effects, depending on the specific proteins targeted.
Preparation Methods
Synthetic Routes and Reaction Conditions
The synthesis of m-PEG20-alcohol typically involves the polymerization of ethylene oxide with methanol as the initiator. The reaction is carried out under alkaline conditions, often using sodium hydroxide as a catalyst. The process results in the formation of a PEG chain with a methoxy group at one end and a hydroxyl group at the other .
Industrial Production Methods
Industrial production of this compound follows similar synthetic routes but on a larger scale. The reaction conditions are optimized to ensure high yield and purity. The product is then purified through processes such as distillation and crystallization to remove any unreacted monomers and by-products .
Chemical Reactions Analysis
Types of Reactions
m-PEG20-alcohol undergoes various chemical reactions, including:
Oxidation: The hydroxyl group can be oxidized to form aldehydes or carboxylic acids.
Reduction: The compound can be reduced to form primary alcohols.
Substitution: The hydroxyl group can be substituted with other functional groups, such as halides or amines.
Common Reagents and Conditions
Oxidation: Common oxidizing agents include potassium permanganate and chromium trioxide.
Reduction: Reducing agents such as lithium aluminum hydride or sodium borohydride are used.
Substitution: Reagents like thionyl chloride or phosphorus tribromide are employed for substitution reactions.
Major Products
Oxidation: Aldehydes or carboxylic acids.
Reduction: Primary alcohols.
Substitution: Halides or amines.
Scientific Research Applications
m-PEG20-alcohol has a wide range of applications in scientific research:
Chemistry: Used as a linker in the synthesis of PROTACs, facilitating the selective degradation of target proteins.
Biology: Employed in the study of protein-protein interactions and cellular pathways.
Comparison with Similar Compounds
Similar Compounds
m-PEG10-alcohol: A shorter PEG chain with similar properties but different molecular weight.
m-PEG30-alcohol: A longer PEG chain with similar properties but different molecular weight.
PEG-diol: A PEG chain with hydroxyl groups at both ends, used in different applications.
Uniqueness
m-PEG20-alcohol is unique due to its specific chain length, which provides an optimal balance between solubility and stability. This makes it particularly suitable for use in PROTACs and other applications where precise control over molecular properties is required .
Biological Activity
m-PEG20-alcohol, a polyethylene glycol (PEG) derivative, has garnered attention in biomedical research due to its potential therapeutic applications, particularly in cancer treatment. This article synthesizes findings from various studies to elucidate the biological activity of this compound, focusing on its pharmacodynamics, pharmacokinetics, and specific case studies that highlight its efficacy.
Overview of this compound
This compound is a mono-PEGylated form of arginine-depleting enzymes, specifically designed to enhance the stability and efficacy of therapeutic agents. PEGylation improves the solubility and bioavailability of drugs while reducing immunogenicity. The 20 kDa PEG moiety significantly alters the pharmacokinetic profile of the conjugated drug.
The primary mechanism by which this compound exerts its biological activity is through the depletion of arginine, an amino acid that is crucial for the growth of certain cancer cells. By reducing arginine levels in the serum, this compound can inhibit the proliferation of arginine-dependent tumors.
Pharmacodynamics
Pharmacodynamic studies have demonstrated that this compound can significantly lower serum L-Arg levels over extended periods. For instance, a study reported that a single intraperitoneal injection of 250 U/mouse resulted in sustained low levels of serum L-Arg for up to 168 hours, compared to only 6 hours with its unmodified counterpart .
Pharmacokinetics
The pharmacokinetic properties of this compound reveal a substantial increase in half-life due to PEGylation. The elimination half-life was extended from approximately 6.4 hours for the unmodified enzyme to 91.4 hours for this compound. This prolonged circulation time enhances therapeutic efficacy and reduces the frequency of administration required .
Efficacy in Cancer Models
-
Lung Cancer Xenograft Model :
- In A549 lung cancer xenograft models, weekly administration of this compound at a dose of 250 U/mouse resulted in significant tumor growth suppression. The IC50 values for various ASS-positive lung cancer cell lines were reported as follows:
Cell Line IC50 (U/mL) A549 2.00 ± 0.99 DMS114 1.49 ± 0.10 NCI-H23 2.11 ± 1.12 NCI-H460 0.81 ± 0.35
- In A549 lung cancer xenograft models, weekly administration of this compound at a dose of 250 U/mouse resulted in significant tumor growth suppression. The IC50 values for various ASS-positive lung cancer cell lines were reported as follows:
-
Safety Profile :
-
Safety evaluations showed no significant adverse effects in normal BALB/c mice following repeated administrations of this compound. Hematological parameters remained stable, with no significant changes observed in white blood cell counts or liver enzyme levels (Table below) .
Parameter PBS Control This compound p Value vs. PBS WBC (K/uL) 2.3 ± 1.2 2.6 ± 1.5 0.802 RBC (M/uL) 8.5 ± 0.3 8.6 ± 0.2 0.622 Hb (g/dL) 13.7 ± 0.6 13.6 ± 0.7 0.825 HCT (%) 46.8 ± 2.1 46.3 ± 1.2 0.676
-
Safety evaluations showed no significant adverse effects in normal BALB/c mice following repeated administrations of this compound. Hematological parameters remained stable, with no significant changes observed in white blood cell counts or liver enzyme levels (Table below) .
Properties
IUPAC Name |
2-[2-[2-[2-[2-[2-[2-[2-[2-[2-[2-[2-[2-[2-[2-[2-[2-[2-[2-(2-methoxyethoxy)ethoxy]ethoxy]ethoxy]ethoxy]ethoxy]ethoxy]ethoxy]ethoxy]ethoxy]ethoxy]ethoxy]ethoxy]ethoxy]ethoxy]ethoxy]ethoxy]ethoxy]ethoxy]ethanol |
Source
|
---|---|---|
Details | Computed by LexiChem 2.6.6 (PubChem release 2019.06.18) | |
Source | PubChem | |
URL | https://pubchem.ncbi.nlm.nih.gov | |
Description | Data deposited in or computed by PubChem | |
InChI |
InChI=1S/C41H84O21/c1-43-4-5-45-8-9-47-12-13-49-16-17-51-20-21-53-24-25-55-28-29-57-32-33-59-36-37-61-40-41-62-39-38-60-35-34-58-31-30-56-27-26-54-23-22-52-19-18-50-15-14-48-11-10-46-7-6-44-3-2-42/h42H,2-41H2,1H3 |
Source
|
Details | Computed by InChI 1.0.5 (PubChem release 2019.06.18) | |
Source | PubChem | |
URL | https://pubchem.ncbi.nlm.nih.gov | |
Description | Data deposited in or computed by PubChem | |
InChI Key |
LBIAEEJHXWQGAM-UHFFFAOYSA-N |
Source
|
Details | Computed by InChI 1.0.5 (PubChem release 2019.06.18) | |
Source | PubChem | |
URL | https://pubchem.ncbi.nlm.nih.gov | |
Description | Data deposited in or computed by PubChem | |
Canonical SMILES |
COCCOCCOCCOCCOCCOCCOCCOCCOCCOCCOCCOCCOCCOCCOCCOCCOCCOCCOCCOCCO |
Source
|
Details | Computed by OEChem 2.1.5 (PubChem release 2019.06.18) | |
Source | PubChem | |
URL | https://pubchem.ncbi.nlm.nih.gov | |
Description | Data deposited in or computed by PubChem | |
Molecular Formula |
C41H84O21 |
Source
|
Details | Computed by PubChem 2.1 (PubChem release 2019.06.18) | |
Source | PubChem | |
URL | https://pubchem.ncbi.nlm.nih.gov | |
Description | Data deposited in or computed by PubChem | |
DSSTOX Substance ID |
DTXSID301025072 |
Source
|
Record name | 2,5,8,11,14,17,20,23,26,29,32,35,38,41,44,47,50,53,56,59-Icosaoxahenhexacontan-61-ol | |
Source | EPA DSSTox | |
URL | https://comptox.epa.gov/dashboard/DTXSID301025072 | |
Description | DSSTox provides a high quality public chemistry resource for supporting improved predictive toxicology. | |
Molecular Weight |
913.1 g/mol |
Source
|
Details | Computed by PubChem 2.1 (PubChem release 2021.05.07) | |
Source | PubChem | |
URL | https://pubchem.ncbi.nlm.nih.gov | |
Description | Data deposited in or computed by PubChem | |
Disclaimer and Information on In-Vitro Research Products
Please be aware that all articles and product information presented on BenchChem are intended solely for informational purposes. The products available for purchase on BenchChem are specifically designed for in-vitro studies, which are conducted outside of living organisms. In-vitro studies, derived from the Latin term "in glass," involve experiments performed in controlled laboratory settings using cells or tissues. It is important to note that these products are not categorized as medicines or drugs, and they have not received approval from the FDA for the prevention, treatment, or cure of any medical condition, ailment, or disease. We must emphasize that any form of bodily introduction of these products into humans or animals is strictly prohibited by law. It is essential to adhere to these guidelines to ensure compliance with legal and ethical standards in research and experimentation.