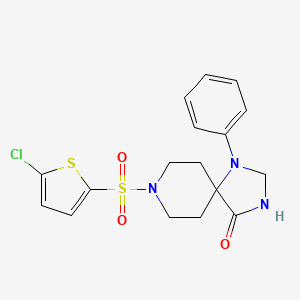
ATP synthase inhibitor 1
Overview
Description
ATP Synthase Inhibitor 1 (CAS 1023043-30-2) is a small-molecule inhibitor targeting the c-subunit of the F1/FO-ATP synthase complex. This compound selectively inhibits the mitochondrial permeability transition pore (mPTP) without affecting ATP synthesis or hydrolysis, making it a unique tool for studying cellular apoptosis and ischemia-reperfusion injury . Its molecular formula is C₁₇H₁₈ClN₃O₃S₂ (molecular weight: 411.93), and it is widely used in preclinical research to explore mitochondrial dysfunction in neurodegenerative and cardiovascular diseases.
Preparation Methods
Synthetic Routes and Reaction Conditions: The synthesis of ATP synthase inhibitor 1 involves multiple steps, including the preparation of precursor molecules and their subsequent chemical modification. The specific synthetic routes and reaction conditions can vary depending on the desired purity and yield of the final product. Common methods include organic synthesis techniques such as nucleophilic substitution, oxidation-reduction reactions, and catalytic hydrogenation .
Industrial Production Methods: Industrial production of this compound typically involves large-scale chemical synthesis using automated reactors and stringent quality control measures. The process may include the use of high-performance liquid chromatography (HPLC) for purification and characterization of the compound. The scalability of the production process ensures a consistent supply of the inhibitor for research and potential therapeutic use .
Chemical Reactions Analysis
Inhibition Mechanism and Binding Dynamics
IF₁ binds to the F₁-ATPase domain via two helices:
-
N-terminal α-helix (residues 8–18) : Inserts into the α/β subunit interface of F₁, blocking catalytic activity .
-
Middle helix (residues 22–50) : Stabilizes the inhibitory conformation through hydrophobic interactions with the γ subunit .
Key Reaction Parameters :
Parameter | Value/Description | Source |
---|---|---|
Optimal inhibitory pH | 6.5–6.7 | |
Binding affinity (Kd) | ~2 nM (pH 6.5) | |
Inhibition efficiency | >95% ATP hydrolysis blockage |
Rotational-Direction-Dependent Activation
IF₁ dissociation is triggered by clockwise rotation (ATP synthesis direction) of the γ subunit in F₁, requiring mechanical force and substrate availability:
Condition | Activation Probability | Substrates Present |
---|---|---|
Clockwise rotation | 60% | ADP + Pi |
Counterclockwise rotation | 0% | ADP + Pi |
Clockwise (no substrates) | 10% | None |
Data derived from single-molecule magnetic tweezers experiments .
Structural Determinants of Inhibition and Release
Mutational Analysis Findings :
IF₁ Variant | Functional Impact |
---|---|
N-terminal truncation | Loss of directional activation |
Middle helix deletion | Reduced inhibition strength |
The γ subunit’s interaction with IF₁’s N-terminal region is critical for force-dependent ejection during ATP synthesis .
pH-Dependent Binding Kinetics
IF₁ binding is enhanced under acidic conditions (e.g., ischemia), protecting cells from ATP depletion:
-
pH 7.4 : Minimal binding (physiological resting state).
Kinetic Parameters :
Process | Time Constant (s) | Conditions |
---|---|---|
IF₁ binding to F₁ | 20–30 | 3 μM IF₁, 1 mM ATP |
IF₁ dissociation | <1 | Clockwise rotation |
Substrate-Specific Reactivation
ATP synthesis substrates (ADP + Pi) facilitate IF₁ dissociation:
Substrate | Reactivation Efficiency |
---|---|
ADP + Pi | 60% |
Pi alone | 28% |
ADP alone | 4% |
This substrate dependency ensures IF₁ inhibition is relieved only when ATP synthesis is thermodynamically favorable .
Pathophysiological Implications
-
Ischemia/reperfusion injury : IF₁ binding at low pH prevents ATP depletion during oxygen deprivation .
-
Cancer metabolism : Overexpression of IF₁ in tumors suppresses ATP hydrolysis, favoring glycolytic metabolism .
IF₁’s conditional inhibition mechanism represents a critical safeguard against cellular energy crises, with therapeutic implications for metabolic disorders and oncology.
Scientific Research Applications
Scientific Research Applications
IF1's functions are linked to tumor formation, progression, and evasion . High expression levels of IF1 in tumor tissues suggest its involvement in cancer . Additionally, IF1 may have protective roles in neurodegenerative and aging-related diseases through its involvement in mitophagy .
Role in Cancer Research
- Target for Anticancer Therapy: High ATP production by mitochondrial ATP synthase is a therapeutic target for anticancer therapy, especially for preventing tumor progression .
- Metastasis Prevention: IF1 may serve as a functional biomarker of metastasis .
- Warburg Effect: IF1 contributes to the Warburg effect, which is a phenomenon where cancer cells preferentially utilize glycolysis over oxidative phosphorylation for energy production .
Study of Mitochondrial Function
- Inhibition of ATP Hydrolysis: IF1 binds to the catalytic domain of ATP synthase during ATP hydrolysis, limiting ATP dissipation under conditions of oxygen deprivation or mitochondrial respiratory complex dysfunction .
- Regulation of ATP Synthase Activity: IF1 regulates the activity of oxidative phosphorylation in mammalian tissues . It is tissue-specifically expressed and primarily controlled at posttranscriptional levels .
IF1 in Diverse Tissues
IF1 content varies among different tissues :
- Heart and Brain: The IF1 content in the heart and brain is four times that of ATP synthase .
- Liver and Kidney: In the liver and kidney, the ATP synthase content exceeds that of IF1 .
Case Studies
- Bedaquiline and Metastasis: The FDA-approved drug Bedaquiline induces ATP depletion in MDA-MB-231 breast cancer cells and prevents spontaneous metastasis in a xenograft model without affecting tumor growth or inducing significant toxicity . Bedaquiline specifically targets ATP5F1C, the gamma-subunit of the mitochondrial ATP synthase .
- IF1 Overexpression and Energy Metabolism: Conditional tissue-specific transgenic mice overexpressing IF1 in the colon, or a mutant active version of IF1 (IF1-H49K) in the liver or neurons, showed inhibition of ATP synthase and reprogramming of energy metabolism to enhanced glycolysis .
- Clinical Significance in Breast Cancer: A study involving 12 female patients with invasive breast carcinoma found a relationship between ATP5F1C protein expression and metastasis . Immunohistochemistry confirmed this relationship .
Data Table: IF1 Expression and Activity
Limitations and Future Directions
Mechanism of Action
ATP synthase inhibitor 1 exerts its effects by binding to specific sites on the ATP synthase enzyme, thereby blocking its activity. This inhibition disrupts the proton gradient across the mitochondrial membrane, preventing the synthesis of ATP from adenosine diphosphate (ADP) and inorganic phosphate. The inhibitor’s binding can also induce conformational changes in the enzyme, further impairing its function .
Comparison with Similar Compounds
Key Compounds and Their Mechanisms
The following table summarizes the structural, functional, and pharmacological differences between ATP Synthase Inhibitor 1 and other ATP synthase inhibitors:
Structural and Functional Insights
- BDQ vs. TBAJ-587: Both diarylquinolines bind to subunit a and the c-ring of Mycobacterium tuberculosis (Mtb) ATP synthase, but TBAJ-587 has a modified side chain that enhances target engagement and reduces off-target effects on human ATP synthase . Cryo-EM studies show BDQ binds to "leading," "c-only," and "lagging" sites in Mtb ATP synthase, while human ATP synthase lacks the "c-only" site, explaining its lower sensitivity .
- This compound vs. IF1 : Unlike the natural peptide IF1, which binds the F1 subunit during ATP hydrolysis, this compound targets the c-subunit and acts independently of ATP levels. IF1’s cardioprotective role involves stabilizing ATP pools during ischemia, whereas Inhibitor 1 prevents mPTP-induced apoptosis .
- Aurovertin and Polyphenols: These inhibitors bind the β-subunit of F1, disrupting rotational catalysis. While aurovertin is non-competitive, polyphenols like resveratrol inhibit via competitive binding to the ATP/ADP site .
Selectivity and Toxicity
Biological Activity
ATP synthase is a crucial enzyme in cellular energy production, responsible for synthesizing adenosine triphosphate (ATP) from adenosine diphosphate (ADP) and inorganic phosphate during oxidative phosphorylation. ATP synthase inhibitor 1 (IF1) is a small protein that plays a significant role in regulating the activity of this enzyme. This article delves into the biological activity of this compound, focusing on its mechanisms of action, physiological implications, and relevance in various pathophysiological conditions.
Inhibition of ATP Synthase Activity
IF1 primarily inhibits the hydrolytic activity of ATP synthase, preventing the enzyme from hydrolyzing ATP back into ADP and inorganic phosphate under certain conditions, particularly during mitochondrial depolarization. This inhibition is crucial for maintaining ATP levels during metabolic stress, such as hypoxia .
Recent studies indicate that IF1 can inhibit both the synthetic and hydrolytic activities of ATP synthase, challenging previous assumptions that it only affects hydrolysis. The phosphorylation state of IF1 is a key regulatory mechanism; for instance, phosphorylation at Ser-14 has been shown to modulate its inhibitory effects .
Tissue-Specific Regulation
The expression and activity of IF1 are tissue-specific and regulated at post-transcriptional levels. In high-energy demanding tissues, such as the heart, IF1 maintains a pool of inactive ATP synthase to facilitate rapid energy demands . Conditional transgenic mouse models have demonstrated that overexpression of IF1 leads to altered energy metabolism, emphasizing its role as a metabolic regulator .
Role in Cancer Metabolism
IF1 has been implicated in cancer biology, where its high expression levels in tumor tissues correlate with enhanced glycolytic metabolism—a hallmark of cancer cells known as the Warburg effect. By inhibiting oxidative phosphorylation (OXPHOS), IF1 favors glycolysis, allowing cancer cells to survive under low oxygen conditions .
Table 1: Summary of IF1's Role in Cancer
Cancer Type | Mechanism | Outcome |
---|---|---|
Osteosarcoma | Inhibition of ATP hydrolysis | Enhanced survival under hypoxia |
Liver Carcinoma | Increased glycolysis | Tumor progression |
Pancreatic Ductal Adenocarcinoma | High IF1/ATP synthase ratio | Maintenance of ATP levels under chemical hypoxia |
Neurodegenerative Diseases
In neurodegenerative contexts, IF1's role appears protective. It may contribute to mitophagy—removal of damaged mitochondria—thereby promoting cell survival under stress conditions . Studies indicate that silencing IF1 in certain cell types leads to increased reactive oxygen species (ROS) production and reduced cell viability under hypoxic conditions .
Conditional Knockout Models
Research utilizing conditional knockout models has provided insights into the physiological roles of IF1. For example, ablation of IF1 in mice resulted in increased ATP production and turnover in mitochondria, confirming its role as an inhibitor under basal OXPHOS conditions . These findings underscore the importance of IF1 in maintaining mitochondrial function and energy homeostasis.
Clinical Observations
Clinical studies have observed elevated levels of IF1 in various cancers, suggesting its potential as a biomarker for tumor progression. For instance, high levels of IF1 have been associated with poor prognosis in patients with liver carcinoma .
Q & A
Basic Research Questions
Q. What experimental methods are commonly used to assess the efficacy and specificity of ATP synthase inhibitors like ATP synthase inhibitor 1?
To evaluate inhibitor efficacy, researchers employ ATPase activity assays using pH-sensitive electrodes to monitor proton flux during ATP hydrolysis (e.g., detecting pH changes via polarography) . For specificity, comparative studies with mitochondrial membrane potential (ΔΨ) assays using TPP+-selective electrodes or fluorometric probes (e.g., rhodamine 123) are recommended to distinguish between direct Fo subunit targeting and off-target effects on proton gradients . Oligomycin, a well-characterized c-ring binder, is often used as a positive control to validate inhibitor mechanisms .
Q. What structural features of ATP synthase are critical for inhibitor binding, and how can these be mapped experimentally?
The c-subunit ring in the Fo domain is a primary target for inhibitors like this compound. Cryo-EM studies of yeast and chloroplast ATP synthase in lipid nanodiscs have resolved inhibitor binding sites (e.g., oligomycin in the c-ring) . Site-directed mutagenesis of conserved residues (e.g., protonatable glutamates in the c-ring) combined with functional assays (e.g., proton translocation measurements) can identify critical binding interfaces .
Q. How does pH influence the activity of ATP synthase inhibitors, and how should this be controlled in experiments?
Inhibitor efficacy can vary with pH due to protonation states of target residues (e.g., glutamate in the c-ring) or regulatory regions of inhibitory proteins. For example, the mitochondrial ATPase inhibitor (IF1) exhibits pH-dependent helicity, with maximal inhibition at pH 6.0–6.5 . Experimental buffers should maintain physiological pH (7.0–7.4) unless studying pH-specific effects, in which case bicarbonate or carbonic anhydrase inhibitors (e.g., acetazolamide) may modulate proton gradients .
Advanced Research Questions
Q. How can conformational heterogeneity in ATP synthase be addressed to improve structural resolution of inhibitor binding modes?
Conformational dynamics pose challenges for cryo-EM studies. Protein engineering (e.g., crosslinking stator and rotor subunits in yeast ATP synthase) or lipid nanodisc reconstitution stabilizes single conformations, enabling high-resolution structural analysis of inhibitor-bound states . Computational methods like molecular dynamics simulations can further predict transient binding pockets .
Q. What strategies resolve contradictions in inhibitor specificity across species (e.g., human vs. M. tuberculosis ATP synthase)?
Comparative cryo-EM of inhibitor-bound complexes (e.g., bedaquiline in human vs. M. tuberculosis ATP synthase) reveals subtle differences in subunit a/c-ring interfaces that dictate species selectivity . Mutagenesis of key residues (e.g., quinolinyl-binding sites in M. tuberculosis) combined with functional assays (e.g., ATP synthesis/hydrolysis rates) can validate mechanistic divergence .
Q. How do researchers differentiate between direct inhibition of ATP synthase and indirect effects on mitochondrial metabolism?
Integrated assays are essential:
- Direct inhibition : Measure oligomycin-sensitive ATPase activity in isolated mitochondria .
- Indirect effects : Combine respirometry (oxygen consumption rates) with ΔΨ measurements to assess uncoupling .
- Metabolic profiling : Compare glycolytic vs. oxidative phosphorylation (OXPHOS) flux via Seahorse assays in inhibitor-treated cells .
Q. What experimental designs address the redox-dependent regulation of ATP synthase activity in inhibitor studies?
Chloroplast ATP synthase has a built-in redox switch (e.g., disulfide bond formation in the γ subunit) that inhibits ATP hydrolysis in the dark . For mitochondrial studies, buffer systems with controlled glutathione ratios (reduced/oxidized) or thioredoxin mimics can modulate redox states . Inhibitor dose-response curves under varying redox conditions (e.g., H2O2 treatment) reveal context-dependent efficacy .
Q. Methodological Notes
- Data contradiction analysis : Conflicting results on inhibitor binding sites may arise from species-specific subunit arrangements (e.g., c-ring stoichiometry: 14 in yeast vs. 8 in mammals) . Cross-validation with orthogonal techniques (e.g., crosslinking mass spectrometry) is critical.
- Advanced tools : Cryo-EM maps (EMDB/PDB), molecular docking (AutoDock), and mitochondrial isolation kits (e.g., differential centrifugation) are recommended for mechanistic studies .
Properties
IUPAC Name |
8-(5-chlorothiophen-2-yl)sulfonyl-1-phenyl-1,3,8-triazaspiro[4.5]decan-4-one | |
---|---|---|
Details | Computed by Lexichem TK 2.7.0 (PubChem release 2021.05.07) | |
Source | PubChem | |
URL | https://pubchem.ncbi.nlm.nih.gov | |
Description | Data deposited in or computed by PubChem | |
InChI |
InChI=1S/C17H18ClN3O3S2/c18-14-6-7-15(25-14)26(23,24)20-10-8-17(9-11-20)16(22)19-12-21(17)13-4-2-1-3-5-13/h1-7H,8-12H2,(H,19,22) | |
Details | Computed by InChI 1.0.6 (PubChem release 2021.05.07) | |
Source | PubChem | |
URL | https://pubchem.ncbi.nlm.nih.gov | |
Description | Data deposited in or computed by PubChem | |
InChI Key |
RYWWSVHLSVFIIG-UHFFFAOYSA-N | |
Details | Computed by InChI 1.0.6 (PubChem release 2021.05.07) | |
Source | PubChem | |
URL | https://pubchem.ncbi.nlm.nih.gov | |
Description | Data deposited in or computed by PubChem | |
Canonical SMILES |
C1CN(CCC12C(=O)NCN2C3=CC=CC=C3)S(=O)(=O)C4=CC=C(S4)Cl | |
Details | Computed by OEChem 2.3.0 (PubChem release 2021.05.07) | |
Source | PubChem | |
URL | https://pubchem.ncbi.nlm.nih.gov | |
Description | Data deposited in or computed by PubChem | |
Molecular Formula |
C17H18ClN3O3S2 | |
Details | Computed by PubChem 2.1 (PubChem release 2021.05.07) | |
Source | PubChem | |
URL | https://pubchem.ncbi.nlm.nih.gov | |
Description | Data deposited in or computed by PubChem | |
Molecular Weight |
411.9 g/mol | |
Details | Computed by PubChem 2.1 (PubChem release 2021.05.07) | |
Source | PubChem | |
URL | https://pubchem.ncbi.nlm.nih.gov | |
Description | Data deposited in or computed by PubChem | |
Retrosynthesis Analysis
AI-Powered Synthesis Planning: Our tool employs the Template_relevance Pistachio, Template_relevance Bkms_metabolic, Template_relevance Pistachio_ringbreaker, Template_relevance Reaxys, Template_relevance Reaxys_biocatalysis model, leveraging a vast database of chemical reactions to predict feasible synthetic routes.
One-Step Synthesis Focus: Specifically designed for one-step synthesis, it provides concise and direct routes for your target compounds, streamlining the synthesis process.
Accurate Predictions: Utilizing the extensive PISTACHIO, BKMS_METABOLIC, PISTACHIO_RINGBREAKER, REAXYS, REAXYS_BIOCATALYSIS database, our tool offers high-accuracy predictions, reflecting the latest in chemical research and data.
Strategy Settings
Precursor scoring | Relevance Heuristic |
---|---|
Min. plausibility | 0.01 |
Model | Template_relevance |
Template Set | Pistachio/Bkms_metabolic/Pistachio_ringbreaker/Reaxys/Reaxys_biocatalysis |
Top-N result to add to graph | 6 |
Feasible Synthetic Routes
Disclaimer and Information on In-Vitro Research Products
Please be aware that all articles and product information presented on BenchChem are intended solely for informational purposes. The products available for purchase on BenchChem are specifically designed for in-vitro studies, which are conducted outside of living organisms. In-vitro studies, derived from the Latin term "in glass," involve experiments performed in controlled laboratory settings using cells or tissues. It is important to note that these products are not categorized as medicines or drugs, and they have not received approval from the FDA for the prevention, treatment, or cure of any medical condition, ailment, or disease. We must emphasize that any form of bodily introduction of these products into humans or animals is strictly prohibited by law. It is essential to adhere to these guidelines to ensure compliance with legal and ethical standards in research and experimentation.