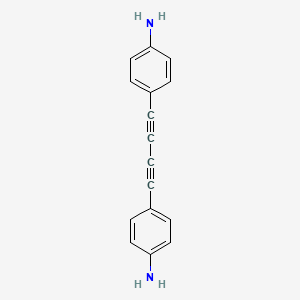
4,4'-(Buta-1,3-diyne-1,4-diyl)dianiline
Overview
Description
4,4'-(Buta-1,3-diyne-1,4-diyl)dianiline (CAS: 30405-78-8), also abbreviated as BDDA, is a symmetric aromatic diamine featuring a central butadiyne (C≡C–C≡C) bridge connecting two para-aminophenyl groups. Its molecular formula is C₁₆H₁₂N₂, with a molecular weight of 232.28 g/mol . The compound is synthesized via copper(II)-catalyzed homocoupling of 4-ethynylaniline, yielding a yellow solid with high purity (84–97%) after column chromatography . Key spectroscopic data includes ¹H NMR (DMSO-d₆, δ 7.20–6.51 ppm for aromatic protons) and MS (ESI) m/z 232.03 (M⁺) . BDDA is soluble in DCM, DMF, and DMSO but requires storage in dark, dry conditions at room temperature .
BDDA serves as a critical building block in covalent organic frameworks (COFs) and pharmaceuticals, particularly as a core scaffold for hepatitis C virus (HCV) NS5A inhibitors .
Preparation Methods
Synthetic Routes and Reaction Conditions
The synthesis of 4,4’-(Buta-1,3-diyne-1,4-diyl)dianiline typically involves the coupling of two aniline derivatives through a butadiyne linkage. One common method involves the use of palladium-catalyzed cross-coupling reactions. For instance, the Sonogashira coupling reaction can be employed, where an aryl halide reacts with a terminal alkyne in the presence of a palladium catalyst and a copper co-catalyst under an inert atmosphere .
Industrial Production Methods
Industrial production of 4,4’-(Buta-1,3-diyne-1,4-diyl)dianiline may involve similar synthetic routes but on a larger scale. The reaction conditions are optimized for higher yields and purity, often involving continuous flow reactors and advanced purification techniques .
Chemical Reactions Analysis
Types of Reactions
4,4’-(Buta-1,3-diyne-1,4-diyl)dianiline undergoes various chemical reactions, including:
Oxidation: The compound can be oxidized to form quinone derivatives.
Reduction: Reduction reactions can convert the butadiyne linkage to a butane linkage.
Substitution: Electrophilic substitution reactions can occur on the aromatic rings.
Common Reagents and Conditions
Oxidation: Common oxidizing agents include potassium permanganate and chromium trioxide.
Reduction: Hydrogenation using palladium on carbon (Pd/C) as a catalyst.
Substitution: Reagents such as bromine or nitric acid can be used for electrophilic aromatic substitution.
Major Products
Oxidation: Quinone derivatives.
Reduction: Butane-linked aniline derivatives.
Substitution: Halogenated or nitrated aniline derivatives.
Scientific Research Applications
Chemical Synthesis and Organic Chemistry
Building Block for Complex Molecules
- 4,4'-(Buta-1,3-diyne-1,4-diyl)dianiline serves as a crucial building block in the synthesis of complex organic molecules. Its structure allows for various functionalizations that are essential in organic synthesis.
- Synthetic Routes : Common methods for synthesizing this compound include palladium-catalyzed cross-coupling reactions like Sonogashira coupling. The reaction typically involves an aryl halide reacting with a terminal alkyne in the presence of a palladium catalyst and a copper co-catalyst under inert conditions.
Reactivity and Functionalization
- The compound can undergo several types of reactions:
- Oxidation : Converts to quinone derivatives using agents like potassium permanganate.
- Reduction : Reduction can yield butane-linked aniline derivatives.
- Substitution : Electrophilic substitution can introduce halogen or nitro groups onto the aromatic rings.
Reaction Type | Example Products |
---|---|
Oxidation | Quinone derivatives |
Reduction | Butane-linked aniline derivatives |
Substitution | Halogenated/nitrated aniline derivatives |
Biological Applications
Biochemical Probes
- Research has explored the potential of this compound as a biochemical probe. Its ability to interact with specific molecular targets makes it suitable for studying enzyme activities and receptor interactions.
Therapeutic Potential
- Preliminary investigations suggest that compounds with similar structures may exhibit antimicrobial or anticancer properties. However, specific biological activity data on this compound remain limited and warrant further study.
Material Science Applications
Conductive Polymers
- The compound is utilized in the production of advanced materials such as conductive polymers and nanomaterials. Its conjugated system imparts interesting electronic properties that are beneficial in developing materials for electronic applications.
Covalent Organic Frameworks (COFs)
- This compound can serve as a monomer in the synthesis of COFs. These frameworks have potential applications in gas storage and catalysis due to their tunable structures and porosities .
Case Study 1: Synthesis of COFs
A recent study demonstrated the use of various monomers including this compound to create COFs with delocalized π-conjugated systems. These materials were characterized for their chemical structures and optical properties, showing promise as photocatalysts for environmental remediation applications .
Mechanism of Action
The mechanism of action of 4,4’-(Buta-1,3-diyne-1,4-diyl)dianiline involves its interaction with specific molecular targets. The butadiyne linkage allows for unique electronic properties, which can influence various biochemical pathways. The compound can interact with enzymes and receptors, modulating their activity and leading to specific biological effects .
Comparison with Similar Compounds
Comparison with Structurally Similar Compounds
Positional Isomer: 3,3'-(Buta-1,3-diyne-1,4-diyl)dianiline
The 3,3'-isomer differs in the substitution pattern of the aniline groups (meta instead of para). This structural variation leads to distinct conformational and electronic properties:
The 3,3'-isomer exhibits superior antiviral activity due to its non-planar conformation, which enhances interactions with the hydrophobic pockets of HCV NS5A . In contrast, the planar structure of BDDA reduces flexibility and binding efficiency .
Functional Analogues in COFs: EDDA vs. BDDA
BDDA is compared with 4,4'-(ethyne-1,2-diyl)dianiline (EDDA) , a COF linker with a single acetylene bridge:
Property | BDDA (Diacetylene) | EDDA (Acetylene) | References |
---|---|---|---|
Photocatalytic HER | 324 ± 5 mmol h⁻¹ g⁻¹ | 30 ± 5 mmol h⁻¹ g⁻¹ | |
Crystallinity | Moderate | High | |
Stability | Stable in acids and water | Similar stability |
The diacetylene bridge in BDDA enhances π-conjugation and charge-carrier mobility, leading to a 10-fold increase in hydrogen evolution rates compared to EDDA .
Substituent Effects on Reactivity
The presence of electron-donating amino groups in BDDA reduces its reactivity in homocoupling reactions compared to nitro- or alkyl-substituted analogues:
Compound | Yield (%) | Reactivity Notes | References |
---|---|---|---|
4,4'-BDDA | 84% | Lower due to amino group | |
1,4-Bis(4-nitrophenyl)butadiyne | 92% | Higher with electron-withdrawing groups |
Electron-withdrawing groups (e.g., nitro) stabilize transition states in coupling reactions, improving yields .
Antiviral Activity
BDDA-based HCV inhibitors exhibit moderate activity, but structural optimization (e.g., imidazole capping) enhances potency:
Compound | Core Scaffold | EC₅₀ (HCV GT1a) | Selectivity Index | |
---|---|---|---|---|
BDDA-amide | 4,4'-BDDA | 4.3 μM | >10³ | |
Compound 10a | 3,3'-BDDA | 0.10 nM | >10⁶ |
The 3,3'-isomer with aromatic capping groups (e.g., phenylalanine) achieves nanomolar potency and broad genotype coverage .
COF Performance
BDDA-derived COFs demonstrate exceptional photocatalytic hydrogen evolution:
COF | Linker | HER Rate (mmol h⁻¹ g⁻¹) | Stability | |
---|---|---|---|---|
TP-BDDA COF | BDDA | 324 ± 5 | Stable in boiling water | |
TP-EDDA COF | EDDA | 30 ± 5 | Similar stability |
The diacetylene moiety in BDDA facilitates efficient light absorption and charge separation .
Biological Activity
4,4'-(Buta-1,3-diyne-1,4-diyl)dianiline (BDDA) is a compound of significant interest due to its unique structural properties and potential biological applications. This article explores the biological activity of BDDA, focusing on its synthesis, mechanisms of action, and relevant case studies.
- Molecular Formula : C₁₆H₁₂N₂
- Molecular Weight : 232.28 g/mol
- CAS Number : 30405-78-8
Synthesis
BDDA can be synthesized through various methods, including microwave-assisted reactions and coupling reactions involving terminal acetylenes. For instance, one study reported the efficient synthesis of BDDA through a homocoupling reaction using copper(I) iodide as a catalyst, yielding high purity products .
Anticancer Properties
BDDA has been investigated for its anticancer properties. In vitro studies have shown that BDDA exhibits cytotoxic effects against various cancer cell lines. The mechanism of action appears to involve the induction of apoptosis and cell cycle arrest. For example, a study demonstrated that BDDA significantly inhibited the proliferation of breast cancer cells by modulating key signaling pathways associated with cell survival and apoptosis .
Antimicrobial Activity
BDDA also displays antimicrobial activity against several bacterial strains. Research has indicated that BDDA can disrupt bacterial cell membranes, leading to cell lysis. A notable study found that BDDA was effective against both Gram-positive and Gram-negative bacteria, suggesting its potential as a broad-spectrum antimicrobial agent .
Photocatalytic Activity
Recent studies have explored the photocatalytic properties of BDDA when incorporated into covalent organic frameworks (COFs). These frameworks demonstrated enhanced hydrogen evolution under light irradiation, indicating that BDDA can serve as an effective component in photocatalytic applications . The presence of diacetylene bridges in COFs significantly improved their photocatalytic efficiency compared to other structures lacking these features.
Case Studies
Study | Findings |
---|---|
Zhang et al., 2022 | Investigated the anticancer effects of BDDA on breast cancer cells; reported significant apoptosis induction and cell cycle arrest. |
Liu et al., 2021 | Explored the antimicrobial efficacy of BDDA; demonstrated broad-spectrum activity against various bacterial strains. |
Thomas et al., 2021 | Analyzed the photocatalytic properties of COFs containing BDDA; found enhanced hydrogen evolution rates under light exposure. |
Q & A
Basic Research Questions
Q. What are the key considerations for synthesizing BDDA with high purity, and how can its structural integrity be validated experimentally?
Methodological Answer: BDDA is synthesized via Sonogashira coupling between 4-iodoaniline and 1,3-butadiyne under palladium catalysis. Critical parameters include:
- Reaction Conditions : Use of anhydrous solvents (e.g., THF), inert atmosphere (N₂/Ar), and controlled temperature (60–80°C) to minimize side reactions .
- Purification : Column chromatography (silica gel, DCM/hexane eluent) removes unreacted monomers and catalysts. Confirm purity via HPLC (C18 column, acetonitrile/water mobile phase) with UV detection at 254 nm .
- Structural Validation :
Q. How does BDDA’s solubility influence its integration into covalent organic frameworks (COFs), and what solvents are optimal for processing?
Methodological Answer: BDDA’s solubility in polar aprotic solvents (DMF, DMSO) enables its use as a linker in COFs. Key steps include:
- Solvent Selection : DMF is preferred for solvothermal COF synthesis due to its high boiling point (153°C) and ability to dissolve BDDA and co-monomers (e.g., triformylphloroglucinol) .
- Crystallinity Optimization : Slow heating (0.5°C/min) to 120°C over 72 hours promotes reversible Schiff-base reactions, ensuring high COF crystallinity .
- Post-Synthesis Processing : Centrifugation (10,000 rpm, 15 min) removes unreacted monomers, followed by Soxhlet extraction with methanol to eliminate residual solvents .
Advanced Research Questions
Q. What mechanistic insights explain BDDA’s role in enhancing photocatalytic hydrogen generation in COFs?
Methodological Answer: BDDA’s diacetylene moiety facilitates charge transfer in COFs. Experimental and computational approaches include:
- Bandgap Engineering : UV-Vis DRS reveals a reduced bandgap (~2.3 eV) in BDDA-based COFs, enabling visible-light absorption. Tauc plots (direct/indirect transitions) correlate with photocatalytic activity .
- Electron Mobility : Time-resolved photoluminescence (TRPL) shows prolonged exciton lifetimes (~5.2 ns), indicating suppressed charge recombination .
- DFT Calculations : HOMO-LUMO analysis (B3LYP/6-31G*) confirms delocalized π-electrons along the diacetylene backbone, enhancing electron-hole separation .
Q. How can researchers resolve contradictions in reported photocatalytic efficiencies of BDDA-based COFs?
Methodological Answer: Discrepancies arise from variations in COF crystallinity, defect density, and testing protocols. Mitigation strategies:
- Standardized Testing : Use a 300 W Xe lamp with AM 1.5G filter (100 mW/cm²) and 10 vol% triethanolamine as a sacrificial agent. Measure H₂ via gas chromatography (TCD detector, Ar carrier) .
- Defect Analysis : Compare PXRD patterns with simulated (Materials Studio) structures; quantify defects via N₂ physisorption (BET surface area < 800 m²/g indicates poor crystallinity) .
- Statistical Design : Apply factorial design (e.g., 2³ matrix) to optimize reaction time, temperature, and monomer ratios, identifying dominant variables via ANOVA .
Q. What advanced spectroscopic techniques characterize BDDA’s electronic interactions in hybrid materials?
Methodological Answer:
- XPS : Analyze N 1s peaks (~399.5 eV for –NH₂) to assess protonation states in acidic environments (e.g., photocatalytic H₂ evolution) .
- In Situ Raman Spectroscopy : Monitor C≡C stretching modes (2150–2200 cm⁻¹) under light irradiation to track structural stability .
- EPR : Detect paramagnetic intermediates (e.g., •OH radicals) using DMPO spin-trapping in aqueous suspensions .
Q. How can BDDA’s stability under operational conditions be systematically evaluated?
Methodological Answer:
- Thermogravimetric Analysis (TGA) : Degradation onset >300°C confirms thermal stability in air/N₂ .
- Cyclic Photocatalysis : Perform 5 consecutive H₂ evolution cycles (4-hour intervals) with intermediate centrifugation/washing. Activity retention <80% indicates structural degradation .
- Accelerated Aging : Expose COFs to UV light (365 nm, 24 hours) and compare PXRD/FTIR data pre-/post-exposure to assess photostability .
Properties
IUPAC Name |
4-[4-(4-aminophenyl)buta-1,3-diynyl]aniline | |
---|---|---|
Details | Computed by Lexichem TK 2.7.0 (PubChem release 2021.05.07) | |
Source | PubChem | |
URL | https://pubchem.ncbi.nlm.nih.gov | |
Description | Data deposited in or computed by PubChem | |
InChI |
InChI=1S/C16H12N2/c17-15-9-5-13(6-10-15)3-1-2-4-14-7-11-16(18)12-8-14/h5-12H,17-18H2 | |
Details | Computed by InChI 1.0.6 (PubChem release 2021.05.07) | |
Source | PubChem | |
URL | https://pubchem.ncbi.nlm.nih.gov | |
Description | Data deposited in or computed by PubChem | |
InChI Key |
AXKIEAUPICVIPV-UHFFFAOYSA-N | |
Details | Computed by InChI 1.0.6 (PubChem release 2021.05.07) | |
Source | PubChem | |
URL | https://pubchem.ncbi.nlm.nih.gov | |
Description | Data deposited in or computed by PubChem | |
Canonical SMILES |
C1=CC(=CC=C1C#CC#CC2=CC=C(C=C2)N)N | |
Details | Computed by OEChem 2.3.0 (PubChem release 2021.05.07) | |
Source | PubChem | |
URL | https://pubchem.ncbi.nlm.nih.gov | |
Description | Data deposited in or computed by PubChem | |
Molecular Formula |
C16H12N2 | |
Details | Computed by PubChem 2.1 (PubChem release 2021.05.07) | |
Source | PubChem | |
URL | https://pubchem.ncbi.nlm.nih.gov | |
Description | Data deposited in or computed by PubChem | |
Molecular Weight |
232.28 g/mol | |
Details | Computed by PubChem 2.1 (PubChem release 2021.05.07) | |
Source | PubChem | |
URL | https://pubchem.ncbi.nlm.nih.gov | |
Description | Data deposited in or computed by PubChem | |
Retrosynthesis Analysis
AI-Powered Synthesis Planning: Our tool employs the Template_relevance Pistachio, Template_relevance Bkms_metabolic, Template_relevance Pistachio_ringbreaker, Template_relevance Reaxys, Template_relevance Reaxys_biocatalysis model, leveraging a vast database of chemical reactions to predict feasible synthetic routes.
One-Step Synthesis Focus: Specifically designed for one-step synthesis, it provides concise and direct routes for your target compounds, streamlining the synthesis process.
Accurate Predictions: Utilizing the extensive PISTACHIO, BKMS_METABOLIC, PISTACHIO_RINGBREAKER, REAXYS, REAXYS_BIOCATALYSIS database, our tool offers high-accuracy predictions, reflecting the latest in chemical research and data.
Strategy Settings
Precursor scoring | Relevance Heuristic |
---|---|
Min. plausibility | 0.01 |
Model | Template_relevance |
Template Set | Pistachio/Bkms_metabolic/Pistachio_ringbreaker/Reaxys/Reaxys_biocatalysis |
Top-N result to add to graph | 6 |
Feasible Synthetic Routes
Disclaimer and Information on In-Vitro Research Products
Please be aware that all articles and product information presented on BenchChem are intended solely for informational purposes. The products available for purchase on BenchChem are specifically designed for in-vitro studies, which are conducted outside of living organisms. In-vitro studies, derived from the Latin term "in glass," involve experiments performed in controlled laboratory settings using cells or tissues. It is important to note that these products are not categorized as medicines or drugs, and they have not received approval from the FDA for the prevention, treatment, or cure of any medical condition, ailment, or disease. We must emphasize that any form of bodily introduction of these products into humans or animals is strictly prohibited by law. It is essential to adhere to these guidelines to ensure compliance with legal and ethical standards in research and experimentation.