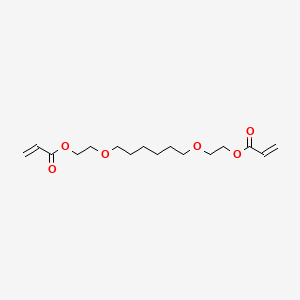
1,6-Hexanediol ethoxylate diacrylate
Overview
Description
1,6-Hexanediol ethoxylate diacrylate is a chemical compound with the molecular formula [H2C=CHCO2(CH2CH2O)nCH2CH2CH2-]2. It is a diacrylate ester of ethoxylated 1,6-hexanediol, commonly used in the production of polymers. This compound is known for its ability to improve the adhesion, hardness, abrasion resistance, and heat resistance of the materials it is incorporated into .
Preparation Methods
1,6-Hexanediol ethoxylate diacrylate is typically synthesized through the esterification of 1,6-hexanediol with acrylic acid in the presence of a catalyst. The process involves the following steps :
Reactants: 1,6-Hexanediol, acrylic acid, solid superacid catalyst, hydroquinone (as a polymerization inhibitor), hypophosphorous acid, and cyclohexane.
Reaction Conditions: The mixture is stirred and heated slowly to a temperature range of 70-80°C, maintaining a steam pressure of about 0.5 Mpa. The reaction is continued at 80-91°C for 9-11 hours.
Post-Reaction: After the reaction, the esterification acid value is checked, and the product is obtained after necessary purification steps.
Chemical Reactions Analysis
1,6-Hexanediol ethoxylate diacrylate undergoes various chemical reactions, including :
Oxidation: Reacts with strong oxidizing agents, potentially forming explosive peroxides.
Reduction: May react exothermically with reducing agents, releasing gaseous hydrogen.
Polymerization: Can undergo addition polymerization reactions, often initiated by free radicals.
Substitution: The hydroxyl groups in the compound can participate in substitution reactions, forming esters and ethers.
Scientific Research Applications
1,6-Hexanediol ethoxylate diacrylate has a wide range of applications in scientific research and industry :
Polymer Synthesis: Used as a monomer for producing various types of polymers, including poly(1,6-hexanediol diacrylate)-based polymer microspheres and bifunctional microcapsules.
Adhesives and Sealants: Enhances adhesion, hardness, and resistance properties in adhesives and sealants.
Coatings and Inks: Improves the performance of alkyd coatings, elastomers, photopolymers, and inks.
Drug Delivery: The resulting polymers can be used in drug delivery systems due to their biocompatibility and controlled release properties.
Sensing and Photonics: Embedded with nanoparticles, these polymers find applications in sensing and photonics.
Mechanism of Action
The mechanism of action of 1,6-Hexanediol ethoxylate diacrylate involves its ability to act as a crosslinking agent between polymer chains . This crosslinking enhances the mechanical properties of the resulting polymers, such as their strength, flexibility, and resistance to environmental factors. The compound’s acrylic functionality allows it to undergo the Michael reaction with amines, which is particularly useful in epoxy chemistry .
Comparison with Similar Compounds
1,6-Hexanediol ethoxylate diacrylate can be compared with other similar compounds, such as :
Trimethylolpropane triacrylate (TMPTA): A triacrylate crosslinker used for similar applications but with three acrylate groups.
Pentaerythritol tetraacrylate: A tetraacrylate crosslinker with four acrylate groups, offering higher crosslinking density.
1,6-Hexanediol diacrylate: The non-ethoxylated version of the compound, used in similar applications but with different properties due to the absence of ethoxy groups.
This compound is unique due to its ethoxylated structure, which provides additional flexibility and compatibility with various polymer matrices.
Properties
IUPAC Name |
2-[6-(2-prop-2-enoyloxyethoxy)hexoxy]ethyl prop-2-enoate | |
---|---|---|
Details | Computed by Lexichem TK 2.7.0 (PubChem release 2021.05.07) | |
Source | PubChem | |
URL | https://pubchem.ncbi.nlm.nih.gov | |
Description | Data deposited in or computed by PubChem | |
InChI |
InChI=1S/C16H26O6/c1-3-15(17)21-13-11-19-9-7-5-6-8-10-20-12-14-22-16(18)4-2/h3-4H,1-2,5-14H2 | |
Details | Computed by InChI 1.0.6 (PubChem release 2021.05.07) | |
Source | PubChem | |
URL | https://pubchem.ncbi.nlm.nih.gov | |
Description | Data deposited in or computed by PubChem | |
InChI Key |
GIMNDOUZTMKUHI-UHFFFAOYSA-N | |
Details | Computed by InChI 1.0.6 (PubChem release 2021.05.07) | |
Source | PubChem | |
URL | https://pubchem.ncbi.nlm.nih.gov | |
Description | Data deposited in or computed by PubChem | |
Canonical SMILES |
C=CC(=O)OCCOCCCCCCOCCOC(=O)C=C | |
Details | Computed by OEChem 2.3.0 (PubChem release 2021.05.07) | |
Source | PubChem | |
URL | https://pubchem.ncbi.nlm.nih.gov | |
Description | Data deposited in or computed by PubChem | |
Molecular Formula |
C16H26O6 | |
Details | Computed by PubChem 2.1 (PubChem release 2021.05.07) | |
Source | PubChem | |
URL | https://pubchem.ncbi.nlm.nih.gov | |
Description | Data deposited in or computed by PubChem | |
DSSTOX Substance ID |
DTXSID701294645 | |
Record name | Diethoxylated hexanediol diacrylate | |
Source | EPA DSSTox | |
URL | https://comptox.epa.gov/dashboard/DTXSID701294645 | |
Description | DSSTox provides a high quality public chemistry resource for supporting improved predictive toxicology. | |
Molecular Weight |
314.37 g/mol | |
Details | Computed by PubChem 2.1 (PubChem release 2021.05.07) | |
Source | PubChem | |
URL | https://pubchem.ncbi.nlm.nih.gov | |
Description | Data deposited in or computed by PubChem | |
CAS No. |
84170-27-4, 226722-38-9 | |
Record name | Ethoxylated 1,6-hexanediol diacrylate | |
Source | CAS Common Chemistry | |
URL | https://commonchemistry.cas.org/detail?cas_rn=84170-27-4 | |
Description | CAS Common Chemistry is an open community resource for accessing chemical information. Nearly 500,000 chemical substances from CAS REGISTRY cover areas of community interest, including common and frequently regulated chemicals, and those relevant to high school and undergraduate chemistry classes. This chemical information, curated by our expert scientists, is provided in alignment with our mission as a division of the American Chemical Society. | |
Explanation | The data from CAS Common Chemistry is provided under a CC-BY-NC 4.0 license, unless otherwise stated. | |
Record name | Diethoxylated hexanediol diacrylate | |
Source | CAS Common Chemistry | |
URL | https://commonchemistry.cas.org/detail?cas_rn=226722-38-9 | |
Description | CAS Common Chemistry is an open community resource for accessing chemical information. Nearly 500,000 chemical substances from CAS REGISTRY cover areas of community interest, including common and frequently regulated chemicals, and those relevant to high school and undergraduate chemistry classes. This chemical information, curated by our expert scientists, is provided in alignment with our mission as a division of the American Chemical Society. | |
Explanation | The data from CAS Common Chemistry is provided under a CC-BY-NC 4.0 license, unless otherwise stated. | |
Record name | Poly(oxy-1,2-ethanediyl), .alpha.,.alpha.'-1,6-hexanediylbis[.omega.-[(1-oxo-2-propen-1-yl)oxy]- | |
Source | EPA Chemicals under the TSCA | |
URL | https://www.epa.gov/chemicals-under-tsca | |
Description | EPA Chemicals under the Toxic Substances Control Act (TSCA) collection contains information on chemicals and their regulations under TSCA, including non-confidential content from the TSCA Chemical Substance Inventory and Chemical Data Reporting. | |
Record name | Diethoxylated hexanediol diacrylate | |
Source | EPA DSSTox | |
URL | https://comptox.epa.gov/dashboard/DTXSID701294645 | |
Description | DSSTox provides a high quality public chemistry resource for supporting improved predictive toxicology. | |
Record name | 2-Propenoic acid, 1,1'-[1,6-hexanediylbis(oxy-2,1-ethanediyl)] ester | |
Source | European Chemicals Agency (ECHA) | |
URL | https://echa.europa.eu/information-on-chemicals | |
Description | The European Chemicals Agency (ECHA) is an agency of the European Union which is the driving force among regulatory authorities in implementing the EU's groundbreaking chemicals legislation for the benefit of human health and the environment as well as for innovation and competitiveness. | |
Explanation | Use of the information, documents and data from the ECHA website is subject to the terms and conditions of this Legal Notice, and subject to other binding limitations provided for under applicable law, the information, documents and data made available on the ECHA website may be reproduced, distributed and/or used, totally or in part, for non-commercial purposes provided that ECHA is acknowledged as the source: "Source: European Chemicals Agency, http://echa.europa.eu/". Such acknowledgement must be included in each copy of the material. ECHA permits and encourages organisations and individuals to create links to the ECHA website under the following cumulative conditions: Links can only be made to webpages that provide a link to the Legal Notice page. | |
Record name | Poly(oxy-1,2-ethanediyl), α,α'-1,6-hexanediylbis[ω-[(1-oxo-2-propen-1-yl)oxy] | |
Source | European Chemicals Agency (ECHA) | |
URL | https://echa.europa.eu/substance-information/-/substanceinfo/100.120.109 | |
Description | The European Chemicals Agency (ECHA) is an agency of the European Union which is the driving force among regulatory authorities in implementing the EU's groundbreaking chemicals legislation for the benefit of human health and the environment as well as for innovation and competitiveness. | |
Explanation | Use of the information, documents and data from the ECHA website is subject to the terms and conditions of this Legal Notice, and subject to other binding limitations provided for under applicable law, the information, documents and data made available on the ECHA website may be reproduced, distributed and/or used, totally or in part, for non-commercial purposes provided that ECHA is acknowledged as the source: "Source: European Chemicals Agency, http://echa.europa.eu/". Such acknowledgement must be included in each copy of the material. ECHA permits and encourages organisations and individuals to create links to the ECHA website under the following cumulative conditions: Links can only be made to webpages that provide a link to the Legal Notice page. | |
Retrosynthesis Analysis
AI-Powered Synthesis Planning: Our tool employs the Template_relevance Pistachio, Template_relevance Bkms_metabolic, Template_relevance Pistachio_ringbreaker, Template_relevance Reaxys, Template_relevance Reaxys_biocatalysis model, leveraging a vast database of chemical reactions to predict feasible synthetic routes.
One-Step Synthesis Focus: Specifically designed for one-step synthesis, it provides concise and direct routes for your target compounds, streamlining the synthesis process.
Accurate Predictions: Utilizing the extensive PISTACHIO, BKMS_METABOLIC, PISTACHIO_RINGBREAKER, REAXYS, REAXYS_BIOCATALYSIS database, our tool offers high-accuracy predictions, reflecting the latest in chemical research and data.
Strategy Settings
Precursor scoring | Relevance Heuristic |
---|---|
Min. plausibility | 0.01 |
Model | Template_relevance |
Template Set | Pistachio/Bkms_metabolic/Pistachio_ringbreaker/Reaxys/Reaxys_biocatalysis |
Top-N result to add to graph | 6 |
Feasible Synthetic Routes
Q1: What makes 1,6-Hexanediol ethoxylate diacrylate suitable for creating monolithic columns for chromatography?
A1: this compound is a cross-linker used in the fabrication of organic monolithic columns for capillary liquid chromatography. [] Its structure, containing two reactive acrylate groups, allows it to form bridges between polymer chains during polymerization. This crosslinking creates a porous, interconnected network within the column. The length of the cross-linker influences the size and distribution of pores, impacting the separation efficiency of the column, particularly for small molecules. [] Researchers have explored its use with monomers like hexyl methacrylate to fine-tune the column's performance. []
Q2: How does varying the type of diacrylate used in poly(β-amino ester) synthesis affect the resulting material?
A2: The choice of diacrylate significantly influences the properties of the resulting poly(β-amino ester) (PBAE) macromers and their subsequent gels. [] Researchers experimented with various diacrylates, including this compound (HDEDA), alongside 1,6-hexanediol diacrylate (HDDA), poly(ethylene glycol) diacrylate (PEGDA), 1,4-butanediol diacrylate (BDDA), and triethylene glycol diacrylate (TEGDA). [] These variations led to differences in molecular weight, degradation rates, and cell interaction behaviors of the PBAE gels. For instance, the HDEDA-based PBAE gel exhibited balanced hydrophilicity, leading to favorable cell attachment compared to some other diacrylate variations. [] This highlights the role of diacrylate structure in tuning material properties for specific biomedical applications.
Q3: Can this compound be used to modify silica for extraction purposes? If so, how?
A3: Yes, this compound, when combined with lauryl methacrylate, can be used to modify silica for extraction applications. [] The process involves synthesizing poly(lauryl methacrylate-co-1,6-hexanediol ethoxylate diacrylate) (LMA-HEDA) directly on silanised silica through free-radical cross-linking polymerization. [] This modification creates a sorbent material suitable for dispersive solid-phase extraction (dSPE). This LMA-HEDA modified silica has shown effectiveness in extracting phenylurea herbicides from environmental water samples. []
Q4: What analytical techniques are employed to characterize materials synthesized with this compound?
A4: Several analytical techniques are used to characterize materials containing this compound. Diffuse Reflectance Fourier Transform Infrared Spectroscopy (DRIFTS) helps analyze the modified silica surface, confirming the presence of the LMA-HEDA polymer. [] Additionally, High-Performance Liquid Chromatography (HPLC) coupled with UV-photodiode array detection allows for the separation and quantification of analytes, like phenylurea herbicides, extracted using the LMA-HEDA modified silica in dSPE. [] These techniques help researchers understand the material's properties and effectiveness in extraction applications.
Disclaimer and Information on In-Vitro Research Products
Please be aware that all articles and product information presented on BenchChem are intended solely for informational purposes. The products available for purchase on BenchChem are specifically designed for in-vitro studies, which are conducted outside of living organisms. In-vitro studies, derived from the Latin term "in glass," involve experiments performed in controlled laboratory settings using cells or tissues. It is important to note that these products are not categorized as medicines or drugs, and they have not received approval from the FDA for the prevention, treatment, or cure of any medical condition, ailment, or disease. We must emphasize that any form of bodily introduction of these products into humans or animals is strictly prohibited by law. It is essential to adhere to these guidelines to ensure compliance with legal and ethical standards in research and experimentation.