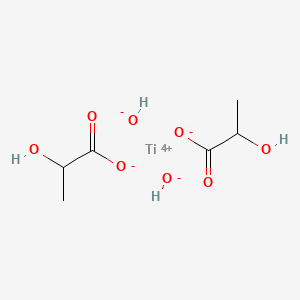
Tilac
Overview
Description
Scientific Research Applications
Tilac has a wide range of scientific research applications, including:
Chemistry: Used as a model compound in the study of NSAIDs and their interactions with biological targets.
Biology: Investigated for its effects on cellular processes and signaling pathways.
Medicine: Extensively studied for its therapeutic effects in treating inflammatory conditions and pain management.
Industry: Utilized in the development of new pharmaceutical formulations and drug delivery systems.
Mechanism of Action
Target of Action
Tilac, developed by TregTherapeutics, is a transformative tolerance induction technology . The primary targets of this compound are the endogenous pathogenic antigens associated with a particular disease . These antigens are responsible for causing the immune response . The approach is highly personalized and targets only the pathogenic self-antigens perpetrating the disease .
Mode of Action
This compound utilizes a formulation comprised of two primary components: a vaccine adjuvant and an anti-inflammatory cytokine . These components have been linked to form a unique conjugate . It accomplishes this with no biological contribution from the patient, such as blood or cells . The approach is simple and elegant, requiring no extracorporeal manipulation of tissue or cellular components .
Biochemical Pathways
The biochemical pathways affected by this compound involve the immune system’s response to endogenous pathogenic antigens . The immune system possesses remarkable restorative power that can be harnessed through the creation and maintenance of a robust tolerogenic microenvironment . This results in directional modulation of the immune response by regulatory T cells to achieve desired disease remediation .
Pharmacokinetics
It is known that both components of the this compound formulation, a vaccine adjuvant and an anti-inflammatory cytokine, are fda-approved and have been safely used clinically for decades .
Result of Action
The result of this compound’s action is the down-regulation of the immune response associated with a particular disease . This is achieved across the range of targeted autoimmune diseases . The immune system’s restorative power is harnessed to create a robust tolerogenic microenvironment, resulting in the directional modulation of the immune response by regulatory T cells .
Action Environment
The action environment of this compound is designed to be a specific, tolerogenic microenvironment . This environment enables a strong and durable outcome of restoration and healing . The approach is considered to be a potentially universal therapy, designed to take advantage of commonality found in all disease states . It is anticipated to be broadly and effectively applied, affecting the lives of millions of people around the world .
Safety and Hazards
Tilac may increase blood pressure and/or cause fluid retention and edema . It also carries a risk of gastrointestinal toxicity, including bleeding, ulceration, and perforation . Other risks include direct renal injury, anaphylactoid reactions, and serious skin reactions . It’s important to use the lowest effective dose for the shortest duration consistent with individual patient treatment goals .
Biochemical Analysis
Biochemical Properties
Tilac leverages nucleoside recoding chemistry to generate characteristic sequencing signatures for each label . It uses statistical modeling to compare the abundance of RNA transcripts between samples . The performance of this compound has been verified in transcriptome-scale experiments involving RNA polymerase II inhibition and heat shock .
Cellular Effects
This compound allows for accurate estimates of differences in RNA levels between experimental and control samples . This is achieved by distinguishing between biological variation and technical noise . The method is particularly useful in advancing biochemical studies, especially in the context of global changes in RNA levels .
Molecular Mechanism
In a this compound experiment, RNA from one sample is labeled with s4U, and the other is labeled with s6G . Cells from each sample are mixed prior to any downstream biochemical steps, like fractionation or polysome purification . During sequencing library preparation, RNA is treated with TimeLapse chemistry to recode the hydrogen bonding pattern of s4U -> C and s6G -> A .
Temporal Effects in Laboratory Settings
This compound is applicable to uncover differences between samples leading to improved biological insights . It can be used to quantify changes in mRNA association with actively translating ribosomes during sodium arsenite stress .
Metabolic Pathways
This compound involves the metabolic labels s4U and s6G . These labels are incorporated into RNA, allowing for the differential labeling of RNAs in cells .
Transport and Distribution
The transport and distribution of this compound within cells and tissues are determined by the incorporation of the metabolic labels into RNA . The labeled RNAs can then be tracked and quantified .
Subcellular Localization
The subcellular localization of this compound is determined by the location of the labeled RNAs. As this compound labels RNA, its localization would be wherever RNA is present within the cell .
Preparation Methods
Synthetic Routes and Reaction Conditions
The synthesis of Tilac involves several steps, starting from the appropriate aromatic and aliphatic precursors. The key steps include:
Aromatic Substitution: The initial step involves the substitution of an aromatic ring with an appropriate aliphatic chain.
Cyclization: The substituted aromatic compound undergoes cyclization to form the core structure of this compound.
Functional Group Modification: The final step involves the modification of functional groups to achieve the desired pharmacological properties.
Industrial Production Methods
Industrial production of this compound typically involves large-scale synthesis using optimized reaction conditions to ensure high yield and purity. The process includes:
Batch Reactors: Utilizing batch reactors for the initial synthesis steps.
Continuous Flow Reactors: Employing continuous flow reactors for the cyclization and functional group modification steps to enhance efficiency and scalability.
Purification: The final product is purified using techniques such as crystallization, filtration, and chromatography to ensure the removal of impurities.
Chemical Reactions Analysis
Types of Reactions
Tilac undergoes several types of chemical reactions, including:
Oxidation: this compound can be oxidized to form various metabolites.
Reduction: Reduction reactions can modify the functional groups on this compound.
Substitution: Substitution reactions can occur on the aromatic ring or aliphatic chain.
Common Reagents and Conditions
Oxidation: Common oxidizing agents include potassium permanganate and hydrogen peroxide.
Reduction: Reducing agents such as sodium borohydride and lithium aluminum hydride are used.
Substitution: Reagents like halogens and alkylating agents are employed for substitution reactions.
Major Products Formed
The major products formed from these reactions include various metabolites and derivatives of this compound, which may have different pharmacological properties.
Comparison with Similar Compounds
Similar Compounds
Ibuprofen: Another NSAID used to treat pain and inflammation.
Naproxen: An NSAID with similar uses but different pharmacokinetic properties.
Meloxicam: A selective COX-2 inhibitor with anti-inflammatory effects.
Uniqueness of Tilac
This compound is unique in its selective inhibition of COX-2, which reduces the risk of gastrointestinal side effects commonly associated with non-selective NSAIDs . Additionally, this compound’s pharmacokinetic profile allows for effective pain management with a lower risk of adverse effects compared to other NSAIDs .
Properties
IUPAC Name |
2-hydroxypropanoate;titanium(4+);dihydroxide | |
---|---|---|
Details | Computed by Lexichem TK 2.7.0 (PubChem release 2021.05.07) | |
Source | PubChem | |
URL | https://pubchem.ncbi.nlm.nih.gov | |
Description | Data deposited in or computed by PubChem | |
InChI |
InChI=1S/2C3H6O3.2H2O.Ti/c2*1-2(4)3(5)6;;;/h2*2,4H,1H3,(H,5,6);2*1H2;/q;;;;+4/p-4 | |
Details | Computed by InChI 1.0.6 (PubChem release 2021.05.07) | |
Source | PubChem | |
URL | https://pubchem.ncbi.nlm.nih.gov | |
Description | Data deposited in or computed by PubChem | |
InChI Key |
DTQHSUHILQWIOM-UHFFFAOYSA-J | |
Details | Computed by InChI 1.0.6 (PubChem release 2021.05.07) | |
Source | PubChem | |
URL | https://pubchem.ncbi.nlm.nih.gov | |
Description | Data deposited in or computed by PubChem | |
Canonical SMILES |
CC(C(=O)[O-])O.CC(C(=O)[O-])O.[OH-].[OH-].[Ti+4] | |
Details | Computed by OEChem 2.3.0 (PubChem release 2021.05.07) | |
Source | PubChem | |
URL | https://pubchem.ncbi.nlm.nih.gov | |
Description | Data deposited in or computed by PubChem | |
Molecular Formula |
C6H12O8Ti | |
Details | Computed by PubChem 2.1 (PubChem release 2021.05.07) | |
Source | PubChem | |
URL | https://pubchem.ncbi.nlm.nih.gov | |
Description | Data deposited in or computed by PubChem | |
Molecular Weight |
260.02 g/mol | |
Details | Computed by PubChem 2.1 (PubChem release 2021.05.07) | |
Source | PubChem | |
URL | https://pubchem.ncbi.nlm.nih.gov | |
Description | Data deposited in or computed by PubChem | |
CAS No. |
79110-90-0 | |
Record name | Titanium, dihydroxybis(2-hydroxypropanoato-O{1},O{2}) | |
Source | European Chemicals Agency (ECHA) | |
URL | https://echa.europa.eu/information-on-chemicals | |
Description | The European Chemicals Agency (ECHA) is an agency of the European Union which is the driving force among regulatory authorities in implementing the EU's groundbreaking chemicals legislation for the benefit of human health and the environment as well as for innovation and competitiveness. | |
Explanation | Use of the information, documents and data from the ECHA website is subject to the terms and conditions of this Legal Notice, and subject to other binding limitations provided for under applicable law, the information, documents and data made available on the ECHA website may be reproduced, distributed and/or used, totally or in part, for non-commercial purposes provided that ECHA is acknowledged as the source: "Source: European Chemicals Agency, http://echa.europa.eu/". Such acknowledgement must be included in each copy of the material. ECHA permits and encourages organisations and individuals to create links to the ECHA website under the following cumulative conditions: Links can only be made to webpages that provide a link to the Legal Notice page. | |
Retrosynthesis Analysis
AI-Powered Synthesis Planning: Our tool employs the Template_relevance Pistachio, Template_relevance Bkms_metabolic, Template_relevance Pistachio_ringbreaker, Template_relevance Reaxys, Template_relevance Reaxys_biocatalysis model, leveraging a vast database of chemical reactions to predict feasible synthetic routes.
One-Step Synthesis Focus: Specifically designed for one-step synthesis, it provides concise and direct routes for your target compounds, streamlining the synthesis process.
Accurate Predictions: Utilizing the extensive PISTACHIO, BKMS_METABOLIC, PISTACHIO_RINGBREAKER, REAXYS, REAXYS_BIOCATALYSIS database, our tool offers high-accuracy predictions, reflecting the latest in chemical research and data.
Strategy Settings
Precursor scoring | Relevance Heuristic |
---|---|
Min. plausibility | 0.01 |
Model | Template_relevance |
Template Set | Pistachio/Bkms_metabolic/Pistachio_ringbreaker/Reaxys/Reaxys_biocatalysis |
Top-N result to add to graph | 6 |
Feasible Synthetic Routes
Disclaimer and Information on In-Vitro Research Products
Please be aware that all articles and product information presented on BenchChem are intended solely for informational purposes. The products available for purchase on BenchChem are specifically designed for in-vitro studies, which are conducted outside of living organisms. In-vitro studies, derived from the Latin term "in glass," involve experiments performed in controlled laboratory settings using cells or tissues. It is important to note that these products are not categorized as medicines or drugs, and they have not received approval from the FDA for the prevention, treatment, or cure of any medical condition, ailment, or disease. We must emphasize that any form of bodily introduction of these products into humans or animals is strictly prohibited by law. It is essential to adhere to these guidelines to ensure compliance with legal and ethical standards in research and experimentation.