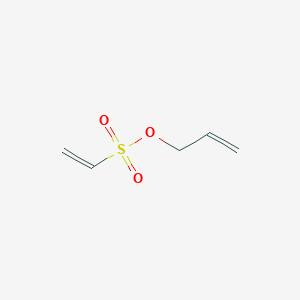
Allyl ethenesulfonate
Overview
Description
Allyl ethenesulfonate (AES) is a sulfonic acid ester. It has been used in polymerization by radical initiators under different conditions . The polymers consist of 67 to 86% of cyclic recurring units for monomer concentrations .
Synthesis Analysis
A practical, highly flexible and eco-friendly method has been developed for the synthesis of allyl thiosulfonates using Morita–Baylis–Hillman (MBH) allyl bromides and sodium arylthiosulfonates . Moreover, the allyl thiosulfonates were successfully transformed into a set of two synthetically viable diallyl disulfanes and unsymmetrical allyl disulfanes in the presence of Cs 2 CO 3 .
Chemical Reactions Analysis
The hydrogen abstraction reaction (HAR) is a major initiation reaction producing primary allyl radicals and leading to chain propagation without degradation chain transfer . These reactions can easily polymerize with free radicals (meth)acrylates or photo-induced auto-oxidation of alkyd resins .
Physical And Chemical Properties Analysis
Sulfonate-based ionic liquids (ILs) with allyl-containing cations have been previously obtained and their thermal, electrochemical and curing properties have been investigated . These ILs present appealing properties, such as low volatility, high thermal stability and high ionic conductivity .
Scientific Research Applications
Polymerization and Copolymerization
Allyl ethenesulfonate (AES) is used in polymerization and copolymerization processes. It has been polymerized using radical initiators under various conditions. The polymers produced consist of a significant percentage of cyclic recurring units. AES exhibits unique reactivity in copolymerization with other monomers like styrene, methyl acrylate, and vinyl acetate, showing different reactivity patterns and resulting in copolymers with varying compositions of cyclic units (Witte & Goethals, 1968).
Surface Passivation in Solar Cells
AES, in the form of methyl/allyl organic monolayers, has been employed in surface passivation for silicon nanowire arrays in hybrid Schottky junction solar cells. This application has led to a significant increase in power conversion efficiency, demonstrating the potential of AES in enhancing the performance of solar cell devices (Zhang et al., 2013).
Influence on Metabolism
AES has been studied for its impact on protein and lipid metabolism. For instance, the effect of AES on the total content and spectrum of proteins and phospholipids in the blood, liver, and kidney tissues has been explored. The studies indicate that AES can cause changes in protein and phospholipid profiles, potentially indicating an adaptive reaction of the organism to the compound (Lubenets et al., 2018).
Antioxidant and Antimicrobial Effects
The antioxidant activities of AES and related compounds have been examined in both in vitro and in vivo settings. These studies have shown significant activities, suggesting a potential role for AES in protecting against oxidative stress. Additionally, allyl-containing compounds have been shown to possess effective antimicrobial properties (Liubas et al., 2023).
Application in Chemistry and Material Science
AES and its derivatives are used in various chemical reactions and material science applications. This includes their use in the synthesis of novel compounds, development of new materials, and in catalytic processes. For instance, allyl esters derived from AES have been used in the preparation of polymers with specific properties (Chang & Miwa, 1979).
Future Directions
Allyl sulfones are excellent reagents for the allylation of radicals with the concomitant elimination of the arylsulfonyl radical as a leaving group . The general reactivity of allyl arylsulfones as radical acceptors usually relies on the attack at the γ-position of the sulfone, promoting a homolytic scission of the C–S via a classical S N 2′ reaction . This suggests potential future directions in the synthesis of allyl sulfones.
Mechanism of Action
Target of Action
Allyl ethenesulfonate, also known as allyl methanesulfonate , is an organic compound that primarily targets alcohols in chemical reactions. It acts as a sulfonylating agent, interacting with alcohols to form sulfonate ester derivatives .
Mode of Action
The compound’s mode of action involves a nucleophilic substitution reaction, similar to the reactions observed with alkyl halides . The key factor in these reactions is the stability of the leaving anion. In the case of this compound, the leaving group is a sulfonate, which is a relatively stable anion. This stability allows the sulfonate group to be replaced by a nucleophile, such as an alcohol .
Biochemical Pathways
The biochemical pathways affected by this compound primarily involve the conversion of alcohols to sulfonate esters . These reactions can lead to the formation of a variety of organosulfur compounds, including thiosulfonates, sulfonamides, sulfides, and sulfones . The compound can also participate in Markovnikov reactions, where it reacts with propenyl alcohol under acidic conditions to form a sulfonate ester .
Pharmacokinetics
Information on the ADME (Absorption, Distribution, Metabolism, and Excretion) properties of this compound is currently limited. It is soluble in alcohols, ethers, and organic solvents, and slightly soluble in water . Its vapor can form explosive mixtures .
Result of Action
The result of this compound’s action is the formation of various organosulfur compounds. These compounds can be used in a variety of applications, including the production of high-performance plastics, rubbers, and coatings . Additionally, the compound can act as a deazotizing agent in organic synthesis reactions .
Action Environment
The action of this compound can be influenced by environmental factors such as temperature and the presence of other reactants. For example, the reaction of this compound with propenyl alcohol to form a sulfonate ester can occur under heated conditions . Additionally, the compound should be handled with appropriate protective equipment in a well-ventilated environment to avoid inhalation of its vapors and to prevent contact with fire sources or static electricity sparks, which could lead to fires or explosions .
properties
IUPAC Name |
prop-2-enyl ethenesulfonate | |
---|---|---|
Details | Computed by LexiChem 2.6.6 (PubChem release 2019.06.18) | |
Source | PubChem | |
URL | https://pubchem.ncbi.nlm.nih.gov | |
Description | Data deposited in or computed by PubChem | |
InChI |
InChI=1S/C5H8O3S/c1-3-5-8-9(6,7)4-2/h3-4H,1-2,5H2 | |
Details | Computed by InChI 1.0.5 (PubChem release 2019.06.18) | |
Source | PubChem | |
URL | https://pubchem.ncbi.nlm.nih.gov | |
Description | Data deposited in or computed by PubChem | |
InChI Key |
UIEFMYGRHVOUTG-UHFFFAOYSA-N | |
Details | Computed by InChI 1.0.5 (PubChem release 2019.06.18) | |
Source | PubChem | |
URL | https://pubchem.ncbi.nlm.nih.gov | |
Description | Data deposited in or computed by PubChem | |
Canonical SMILES |
C=CCOS(=O)(=O)C=C | |
Details | Computed by OEChem 2.1.5 (PubChem release 2019.06.18) | |
Source | PubChem | |
URL | https://pubchem.ncbi.nlm.nih.gov | |
Description | Data deposited in or computed by PubChem | |
Molecular Formula |
C5H8O3S | |
Details | Computed by PubChem 2.1 (PubChem release 2019.06.18) | |
Source | PubChem | |
URL | https://pubchem.ncbi.nlm.nih.gov | |
Description | Data deposited in or computed by PubChem | |
Molecular Weight |
148.18 g/mol | |
Details | Computed by PubChem 2.1 (PubChem release 2021.05.07) | |
Source | PubChem | |
URL | https://pubchem.ncbi.nlm.nih.gov | |
Description | Data deposited in or computed by PubChem | |
Retrosynthesis Analysis
AI-Powered Synthesis Planning: Our tool employs the Template_relevance Pistachio, Template_relevance Bkms_metabolic, Template_relevance Pistachio_ringbreaker, Template_relevance Reaxys, Template_relevance Reaxys_biocatalysis model, leveraging a vast database of chemical reactions to predict feasible synthetic routes.
One-Step Synthesis Focus: Specifically designed for one-step synthesis, it provides concise and direct routes for your target compounds, streamlining the synthesis process.
Accurate Predictions: Utilizing the extensive PISTACHIO, BKMS_METABOLIC, PISTACHIO_RINGBREAKER, REAXYS, REAXYS_BIOCATALYSIS database, our tool offers high-accuracy predictions, reflecting the latest in chemical research and data.
Strategy Settings
Precursor scoring | Relevance Heuristic |
---|---|
Min. plausibility | 0.01 |
Model | Template_relevance |
Template Set | Pistachio/Bkms_metabolic/Pistachio_ringbreaker/Reaxys/Reaxys_biocatalysis |
Top-N result to add to graph | 6 |
Feasible Synthetic Routes
Disclaimer and Information on In-Vitro Research Products
Please be aware that all articles and product information presented on BenchChem are intended solely for informational purposes. The products available for purchase on BenchChem are specifically designed for in-vitro studies, which are conducted outside of living organisms. In-vitro studies, derived from the Latin term "in glass," involve experiments performed in controlled laboratory settings using cells or tissues. It is important to note that these products are not categorized as medicines or drugs, and they have not received approval from the FDA for the prevention, treatment, or cure of any medical condition, ailment, or disease. We must emphasize that any form of bodily introduction of these products into humans or animals is strictly prohibited by law. It is essential to adhere to these guidelines to ensure compliance with legal and ethical standards in research and experimentation.