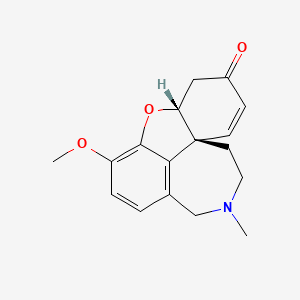
(+)-Narwedine
Overview
Description
(+)-Narwedine: is a naturally occurring alkaloid found in certain plant species. It is a chiral compound, meaning it has a specific three-dimensional arrangement that distinguishes it from its mirror image. This compound is of significant interest due to its potential pharmacological properties and its role as a precursor in the synthesis of other biologically active molecules.
Preparation Methods
Synthetic Routes and Reaction Conditions: The synthesis of (+)-Narwedine typically involves several steps, starting from simpler organic molecules. One common synthetic route includes the use of a chiral auxiliary to induce the desired stereochemistry. The process often involves:
Formation of an intermediate: This step may involve the use of reagents such as lithium diisopropylamide (LDA) to form an enolate, which then reacts with an electrophile to form a key intermediate.
Cyclization: The intermediate undergoes a cyclization reaction, often facilitated by a Lewis acid such as titanium tetrachloride, to form the core structure of this compound.
Final adjustments: The final steps may include reduction or oxidation reactions to achieve the desired functional groups and stereochemistry.
Industrial Production Methods: Industrial production of this compound may involve similar synthetic routes but optimized for large-scale production. This includes the use of continuous flow reactors to improve reaction efficiency and yield. Additionally, the use of green chemistry principles, such as solvent recycling and minimizing waste, is often emphasized in industrial settings.
Chemical Reactions Analysis
Types of Reactions:
Oxidation: (+)-Narwedine can undergo oxidation reactions to form various oxidized derivatives. Common oxidizing agents include potassium permanganate and chromium trioxide.
Reduction: Reduction reactions can convert this compound into its reduced forms. Sodium borohydride and lithium aluminum hydride are typical reducing agents used.
Substitution: The compound can participate in substitution reactions, where functional groups are replaced by other groups. This can be achieved using reagents like alkyl halides or acyl chlorides.
Common Reagents and Conditions:
Oxidation: Potassium permanganate in an acidic medium.
Reduction: Sodium borohydride in methanol.
Substitution: Alkyl halides in the presence of a base such as sodium hydroxide.
Major Products Formed:
Oxidation: Oxidized derivatives with additional oxygen-containing functional groups.
Reduction: Reduced forms with fewer oxygen-containing functional groups.
Substitution: Substituted derivatives with new functional groups replacing the original ones.
Scientific Research Applications
Chemistry: : (+)-Narwedine is used as a precursor in the synthesis of other complex organic molecules. Its unique structure makes it a valuable starting material for the development of new synthetic methodologies.
Biology: : In biological research, this compound is studied for its potential effects on various biological pathways. Its interactions with enzymes and receptors are of particular interest.
Medicine: : The compound is being investigated for its potential therapeutic properties. Preliminary studies suggest it may have applications in treating certain neurological disorders.
Industry: : In the industrial sector, this compound is used in the production of fine chemicals and pharmaceuticals. Its role as an intermediate in the synthesis of other valuable compounds makes it an important chemical in industrial processes.
Mechanism of Action
The mechanism of action of (+)-Narwedine involves its interaction with specific molecular targets in the body. It is believed to exert its effects by binding to certain enzymes or receptors, thereby modulating their activity. The exact pathways involved are still under investigation, but it is thought to influence neurotransmitter systems and other cellular processes.
Comparison with Similar Compounds
Similar Compounds
(-)-Narwedine: The enantiomer of (+)-Narwedine, with a mirror-image structure.
Galantamine: A related alkaloid with similar pharmacological properties.
Codeine: Another alkaloid with a similar core structure but different functional groups.
Uniqueness: : this compound is unique due to its specific stereochemistry, which can result in different biological activities compared to its enantiomer and other similar compounds. Its role as a precursor in the synthesis of other biologically active molecules also sets it apart from related compounds.
Properties
IUPAC Name |
(1R,12R)-9-methoxy-4-methyl-11-oxa-4-azatetracyclo[8.6.1.01,12.06,17]heptadeca-6(17),7,9,15-tetraen-14-one | |
---|---|---|
Details | Computed by Lexichem TK 2.7.0 (PubChem release 2021.05.07) | |
Source | PubChem | |
URL | https://pubchem.ncbi.nlm.nih.gov | |
Description | Data deposited in or computed by PubChem | |
InChI |
InChI=1S/C17H19NO3/c1-18-8-7-17-6-5-12(19)9-14(17)21-16-13(20-2)4-3-11(10-18)15(16)17/h3-6,14H,7-10H2,1-2H3/t14-,17-/m1/s1 | |
Details | Computed by InChI 1.0.6 (PubChem release 2021.05.07) | |
Source | PubChem | |
URL | https://pubchem.ncbi.nlm.nih.gov | |
Description | Data deposited in or computed by PubChem | |
InChI Key |
QENVUHCAYXAROT-RHSMWYFYSA-N | |
Details | Computed by InChI 1.0.6 (PubChem release 2021.05.07) | |
Source | PubChem | |
URL | https://pubchem.ncbi.nlm.nih.gov | |
Description | Data deposited in or computed by PubChem | |
Canonical SMILES |
CN1CCC23C=CC(=O)CC2OC4=C(C=CC(=C34)C1)OC | |
Details | Computed by OEChem 2.3.0 (PubChem release 2021.05.07) | |
Source | PubChem | |
URL | https://pubchem.ncbi.nlm.nih.gov | |
Description | Data deposited in or computed by PubChem | |
Isomeric SMILES |
CN1CC[C@]23C=CC(=O)C[C@H]2OC4=C(C=CC(=C34)C1)OC | |
Details | Computed by OEChem 2.3.0 (PubChem release 2021.05.07) | |
Source | PubChem | |
URL | https://pubchem.ncbi.nlm.nih.gov | |
Description | Data deposited in or computed by PubChem | |
Molecular Formula |
C17H19NO3 | |
Details | Computed by PubChem 2.1 (PubChem release 2021.05.07) | |
Source | PubChem | |
URL | https://pubchem.ncbi.nlm.nih.gov | |
Description | Data deposited in or computed by PubChem | |
Molecular Weight |
285.34 g/mol | |
Details | Computed by PubChem 2.1 (PubChem release 2021.05.07) | |
Source | PubChem | |
URL | https://pubchem.ncbi.nlm.nih.gov | |
Description | Data deposited in or computed by PubChem | |
CAS No. |
7318-55-0 | |
Record name | Narwedine, (+)- | |
Source | ChemIDplus | |
URL | https://pubchem.ncbi.nlm.nih.gov/substance/?source=chemidplus&sourceid=0007318550 | |
Description | ChemIDplus is a free, web search system that provides access to the structure and nomenclature authority files used for the identification of chemical substances cited in National Library of Medicine (NLM) databases, including the TOXNET system. | |
Record name | NARWEDINE, (+)- | |
Source | FDA Global Substance Registration System (GSRS) | |
URL | https://gsrs.ncats.nih.gov/ginas/app/beta/substances/6A3S1D0Z5T | |
Description | The FDA Global Substance Registration System (GSRS) enables the efficient and accurate exchange of information on what substances are in regulated products. Instead of relying on names, which vary across regulatory domains, countries, and regions, the GSRS knowledge base makes it possible for substances to be defined by standardized, scientific descriptions. | |
Explanation | Unless otherwise noted, the contents of the FDA website (www.fda.gov), both text and graphics, are not copyrighted. They are in the public domain and may be republished, reprinted and otherwise used freely by anyone without the need to obtain permission from FDA. Credit to the U.S. Food and Drug Administration as the source is appreciated but not required. | |
Retrosynthesis Analysis
AI-Powered Synthesis Planning: Our tool employs the Template_relevance Pistachio, Template_relevance Bkms_metabolic, Template_relevance Pistachio_ringbreaker, Template_relevance Reaxys, Template_relevance Reaxys_biocatalysis model, leveraging a vast database of chemical reactions to predict feasible synthetic routes.
One-Step Synthesis Focus: Specifically designed for one-step synthesis, it provides concise and direct routes for your target compounds, streamlining the synthesis process.
Accurate Predictions: Utilizing the extensive PISTACHIO, BKMS_METABOLIC, PISTACHIO_RINGBREAKER, REAXYS, REAXYS_BIOCATALYSIS database, our tool offers high-accuracy predictions, reflecting the latest in chemical research and data.
Strategy Settings
Precursor scoring | Relevance Heuristic |
---|---|
Min. plausibility | 0.01 |
Model | Template_relevance |
Template Set | Pistachio/Bkms_metabolic/Pistachio_ringbreaker/Reaxys/Reaxys_biocatalysis |
Top-N result to add to graph | 6 |
Feasible Synthetic Routes
Disclaimer and Information on In-Vitro Research Products
Please be aware that all articles and product information presented on BenchChem are intended solely for informational purposes. The products available for purchase on BenchChem are specifically designed for in-vitro studies, which are conducted outside of living organisms. In-vitro studies, derived from the Latin term "in glass," involve experiments performed in controlled laboratory settings using cells or tissues. It is important to note that these products are not categorized as medicines or drugs, and they have not received approval from the FDA for the prevention, treatment, or cure of any medical condition, ailment, or disease. We must emphasize that any form of bodily introduction of these products into humans or animals is strictly prohibited by law. It is essential to adhere to these guidelines to ensure compliance with legal and ethical standards in research and experimentation.