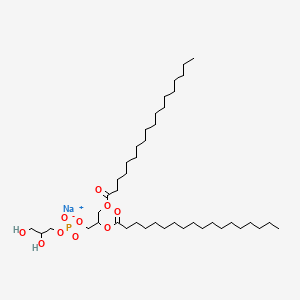
Mpeg-dspe
Overview
Description
Methoxypolyethylene glycol DSPE (mPEG-DSPE) is a phospholipid PEG derivative that has a terminal DSPE group on the PEG end . DSPE (1,2-distearoyl-sn-glycero-3-phospho-ethanolamine) is a C18 saturated phospholipid with high hydrophobicity . It demonstrates excellent amphiphilic properties and offers superior advantages for small and large molecule encapsulation and delivery .
Synthesis Analysis
The synthesis of this compound generally involves two steps :
- An amidation reaction is carried out by adding an appropriate amount of acetamide and a base to produce this compound .
Molecular Structure Analysis
The molecular formula of this compound is C37H72O12PN . The molecular weight is approximately 746.94g/mol .
Chemical Reactions Analysis
This compound is used in the synthesis of liposomes, which increases the hydrophilicity on the surface of liposomes, consequently increasing the longevity of liposomes in the blood circulation for the study of anticancer and antimalarial agents .
Physical and Chemical Properties Analysis
This compound appears as a white to off-white solid or viscous liquid depending on the molecular weight . It has a melting point of about 50-60 ℃ . It is soluble in inorganic solvents and organic solvents, such as chloroform, methanol, dimethyl sulfoxide, etc .
Scientific Research Applications
MPEG-DSPE in Nanoparticle Imaging and Drug Delivery
This compound has been utilized in the development of near-infrared fluorophores for skeletal system imaging. A study highlighted the use of DSPE-mPEG encapsulated rare earth doped nanoparticles (RENPs@DSPE-mPEG) demonstrating an inherent affinity to bone without targeting ligands. This approach offers a non-invasive strategy for skeletal system mapping and bone disease diagnoses, with potential in immunotherapy and cancer-targeted nanoparticles (He et al., 2019).
Impact on Liposomal Drug Formulations
DSPE-mPEG, a family of amphiphilic lipopolymers, plays a significant role in formulating injectable, long-circulating nanoparticulate drug formulations. Research has shown its effectiveness in PEGylation of lipidic nonlamellar liquid crystalline particles, which are used in developing cubosomes and hexosomes for drug and imaging probe delivery. The incorporation of DSPE-mPEG into these formulations impacts their morphology and internal nanostructure, enhancing their potential in drug delivery systems (Nilsson et al., 2014).
Reversible Attachment of Polyethylene Glycol
The reversible attachment of methoxypoly(ethylene glycol) (mPEG) to amino-containing substrates using DSPE has been explored. This strategy, involving the formation of a benzyl carbamate linkage substituted with a disulfide, allows the creation of lipopolymers capable of losing their polymer coating under specific conditions. This approach is significant in the context of drug delivery, where controlled release and targeting are crucial (Zalipsky et al., 1999).
Cell Surface Engineering
DSPE-mPEG has been investigated in the context of cell surface engineering. It has been used to modify the cell surface, impacting various cellular behaviors such as viability, growth, proliferation, and adhesion. This study demonstrated that DSPE-mPEG modification highly affects cell adherence, providing insights into the application of cell surface engineering in biomedicine (Cai et al., 2022).
Mechanism of Action
Target of Action
MPEG-DSPE is a phospholipid polyethylene glycol (PEG) derivative that has a terminal distearoylphosphatidylethanolamine (DSPE) group on the PEG end . The primary targets of this compound are cell membranes and liposomes, where it is used for drug formulation, cell membrane study, and other studies .
Mode of Action
This compound interacts with its targets by integrating into the lipid bilayer of cell membranes or liposomes. The DSPE component, a C18 saturated phospholipid with high hydrophobicity, anchors the molecule in the lipid bilayer, while the PEG component extends outwards, providing a hydrophilic surface . This amphiphilic property allows this compound to enhance the water solubility and stability of molecules, particles, and materials, reduce their immunogenicity, and decrease non-specific binding .
Biochemical Pathways
This compound does not directly affect specific biochemical pathways. Instead, it modifies the physicochemical properties of molecules, particles, and materials, thereby influencing their interactions with biological systems. For instance, this compound can be used to form nanoparticles with enhanced water solubility and biocompatibility , which can affect various biological processes depending on the specific molecules or particles encapsulated.
Pharmacokinetics
The pharmacokinetics of this compound largely depends on its application. When used to form long-circulating liposomes, this compound can prolong the circulation time of the liposomes in the bloodstream . This is because the PEGylation of DSPE reduces the recognition and uptake of the liposomes by the mononuclear phagocyte system (MPS), thereby enhancing their bioavailability .
Result of Action
The effects of this compound are primarily related to its ability to modify the properties of molecules, particles, and materials. For example, when used to form nanoparticles, this compound can enhance their water solubility and biocompatibility . This can lead to various molecular and cellular effects, depending on the specific molecules or particles encapsulated. In one study, this compound was used to form nanoparticles with corannulene or perylene, which were found to affect the first polar body extrusion of oocytes, increase the number of primordial follicles in the ovary, alter mitochondrial membrane potentials, induce oxidative stress, and lead to autophagy and apoptosis .
Action Environment
The action of this compound can be influenced by various environmental factors. For instance, the stability of this compound can be affected by temperature, as it should always be kept in low temperature in dry condition . Moreover, the efficacy of this compound in forming long-circulating liposomes can be influenced by the properties of the biological environment, such as the presence of MPS-rich tissues .
Future Directions
Biochemical Analysis
Biochemical Properties
Mpeg-dspe plays a crucial role in biochemical reactions. It is used as a marker in biological analysis, such as biological imaging, immunoassay, and fluorescent probes . It interacts with various enzymes, proteins, and other biomolecules, and the nature of these interactions is primarily through the formation of lipid nanoparticles (LNPs) .
Cellular Effects
This compound has significant effects on various types of cells and cellular processes. It influences cell function by modulating particle size and increasing stability, thereby decreasing particle aggregation and increasing circulation time . This can impact cell signaling pathways, gene expression, and cellular metabolism.
Molecular Mechanism
The mechanism of action of this compound is complex. It exerts its effects at the molecular level primarily through the formation of LNPs, which are used as drug delivery vehicles . These LNPs can bind to biomolecules, inhibit or activate enzymes, and cause changes in gene expression .
Metabolic Pathways
This compound is involved in various metabolic pathways. It interacts with enzymes or cofactors, and can affect metabolic flux or metabolite levels
Transport and Distribution
This compound is transported and distributed within cells and tissues. It can interact with transporters or binding proteins, and can affect its localization or accumulation
Properties
IUPAC Name |
sodium;2,3-dihydroxypropyl 2,3-di(octadecanoyloxy)propyl phosphate | |
---|---|---|
Details | Computed by Lexichem TK 2.7.0 (PubChem release 2021.05.07) | |
Source | PubChem | |
URL | https://pubchem.ncbi.nlm.nih.gov | |
Description | Data deposited in or computed by PubChem | |
InChI |
InChI=1S/C42H83O10P.Na/c1-3-5-7-9-11-13-15-17-19-21-23-25-27-29-31-33-41(45)49-37-40(38-51-53(47,48)50-36-39(44)35-43)52-42(46)34-32-30-28-26-24-22-20-18-16-14-12-10-8-6-4-2;/h39-40,43-44H,3-38H2,1-2H3,(H,47,48);/q;+1/p-1 | |
Details | Computed by InChI 1.0.6 (PubChem release 2021.05.07) | |
Source | PubChem | |
URL | https://pubchem.ncbi.nlm.nih.gov | |
Description | Data deposited in or computed by PubChem | |
InChI Key |
YNQYZBDRJZVSJE-UHFFFAOYSA-M | |
Details | Computed by InChI 1.0.6 (PubChem release 2021.05.07) | |
Source | PubChem | |
URL | https://pubchem.ncbi.nlm.nih.gov | |
Description | Data deposited in or computed by PubChem | |
Canonical SMILES |
CCCCCCCCCCCCCCCCCC(=O)OCC(COP(=O)([O-])OCC(CO)O)OC(=O)CCCCCCCCCCCCCCCCC.[Na+] | |
Details | Computed by OEChem 2.3.0 (PubChem release 2021.05.07) | |
Source | PubChem | |
URL | https://pubchem.ncbi.nlm.nih.gov | |
Description | Data deposited in or computed by PubChem | |
Molecular Formula |
C42H82NaO10P | |
Details | Computed by PubChem 2.1 (PubChem release 2021.05.07) | |
Source | PubChem | |
URL | https://pubchem.ncbi.nlm.nih.gov | |
Description | Data deposited in or computed by PubChem | |
Molecular Weight |
801.1 g/mol | |
Details | Computed by PubChem 2.1 (PubChem release 2021.05.07) | |
Source | PubChem | |
URL | https://pubchem.ncbi.nlm.nih.gov | |
Description | Data deposited in or computed by PubChem | |
Retrosynthesis Analysis
AI-Powered Synthesis Planning: Our tool employs the Template_relevance Pistachio, Template_relevance Bkms_metabolic, Template_relevance Pistachio_ringbreaker, Template_relevance Reaxys, Template_relevance Reaxys_biocatalysis model, leveraging a vast database of chemical reactions to predict feasible synthetic routes.
One-Step Synthesis Focus: Specifically designed for one-step synthesis, it provides concise and direct routes for your target compounds, streamlining the synthesis process.
Accurate Predictions: Utilizing the extensive PISTACHIO, BKMS_METABOLIC, PISTACHIO_RINGBREAKER, REAXYS, REAXYS_BIOCATALYSIS database, our tool offers high-accuracy predictions, reflecting the latest in chemical research and data.
Strategy Settings
Precursor scoring | Relevance Heuristic |
---|---|
Min. plausibility | 0.01 |
Model | Template_relevance |
Template Set | Pistachio/Bkms_metabolic/Pistachio_ringbreaker/Reaxys/Reaxys_biocatalysis |
Top-N result to add to graph | 6 |
Feasible Synthetic Routes
A: Mpeg-dspe is an amphiphilic molecule, meaning it possesses both hydrophilic (water-loving) and hydrophobic (water-fearing) regions. The polyethylene glycol (PEG) chain provides the hydrophilic character, while the distearoylphosphatidylethanolamine (DSPE) portion contributes to the hydrophobic nature. This unique characteristic allows this compound to self-assemble into micelles in aqueous solutions, with the hydrophobic drug molecules encapsulated within the hydrophobic core and the hydrophilic PEG chains forming the outer shell. [, ] This encapsulation shields the drug from the external environment, improving its solubility and stability in aqueous media, and enabling its delivery to target sites.
A: this compound is commonly incorporated into liposomal formulations to enhance their stability and circulation time in vivo. The hydrophilic PEG chains on the liposome surface create a steric barrier that hinders interactions with blood components and reduces uptake by the reticuloendothelial system (RES), leading to prolonged circulation and improved drug delivery to target tissues. [, ]
A: Yes, this compound can be conjugated with targeting ligands, such as folate or peptides, to enhance drug delivery to specific cells or tissues. For instance, folate-conjugated this compound micelles have been explored for targeted delivery of anticancer drugs to tumor cells overexpressing folate receptors. [, , ] Similarly, peptide-modified this compound micelles have shown promise in delivering drugs to specific receptors overexpressed on cancer cells. []
A: this compound-based formulations, like liposomes and micelles, exhibit good stability under various storage conditions. Lyophilization with suitable cryoprotectants, such as sucrose, further enhances their shelf life. [, ]
A: Factors like temperature, pH, ionic strength, and the presence of enzymes can impact the stability of this compound formulations. Optimization of these parameters during formulation development is crucial to ensure long-term stability and therapeutic efficacy. [, ]
A: In vitro studies evaluating this compound-based systems often involve cell culture models to assess drug release profiles, cellular uptake, cytotoxicity, and targeting efficiency. [, , ] For instance, fluorescence microscopy has been used to investigate the cellular uptake of fluorescently labeled this compound micelles. [, ]
A: In vivo studies utilizing this compound formulations often employ animal models to evaluate pharmacokinetic parameters, biodistribution, tumor targeting, and therapeutic efficacy. [, ] For example, studies in mice have demonstrated enhanced antitumor activity of paclitaxel and doxorubicin when encapsulated in this compound-based delivery systems. [, ]
Disclaimer and Information on In-Vitro Research Products
Please be aware that all articles and product information presented on BenchChem are intended solely for informational purposes. The products available for purchase on BenchChem are specifically designed for in-vitro studies, which are conducted outside of living organisms. In-vitro studies, derived from the Latin term "in glass," involve experiments performed in controlled laboratory settings using cells or tissues. It is important to note that these products are not categorized as medicines or drugs, and they have not received approval from the FDA for the prevention, treatment, or cure of any medical condition, ailment, or disease. We must emphasize that any form of bodily introduction of these products into humans or animals is strictly prohibited by law. It is essential to adhere to these guidelines to ensure compliance with legal and ethical standards in research and experimentation.