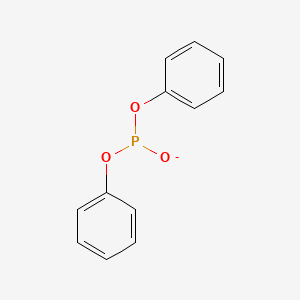
Diphenoxyphosphanolate
Overview
Description
Diphenoxyphosphanolate is a synthetic organic compound with the molecular formula C12H10O3P. . This compound is part of the broader class of phosphonates, which are known for their diverse chemical properties and biological activities.
Scientific Research Applications
Diphenoxyphosphanolate has a wide range of applications in scientific research:
Chemistry: It is used as a reagent in organic synthesis, particularly in the formation of C-P bonds.
Biology: Its derivatives are studied for their potential as enzyme inhibitors and antimicrobial agents.
Medicine: Research is ongoing into its potential use in drug development, particularly for antiviral and anticancer therapies.
Industry: It is utilized in the production of flame retardants and plasticizers
Preparation Methods
Synthetic Routes and Reaction Conditions: Diphenoxyphosphanolate can be synthesized through various methods. One common approach involves the reaction of diphenylphosphine oxide with phenol in the presence of a base. This reaction typically occurs under mild conditions and yields this compound as the primary product .
Industrial Production Methods: Industrial production of this compound often employs large-scale batch reactors where the reaction conditions are carefully controlled to optimize yield and purity. The use of catalysts, such as palladium or copper, can enhance the efficiency of the synthesis process .
Chemical Reactions Analysis
Types of Reactions: Diphenoxyphosphanolate undergoes various chemical reactions, including:
Oxidation: It can be oxidized to form phosphonic acids.
Reduction: Reduction reactions can convert it into phosphine derivatives.
Substitution: It participates in nucleophilic substitution reactions, where the phenoxy groups can be replaced by other nucleophiles.
Common Reagents and Conditions:
Oxidation: Common oxidizing agents include hydrogen peroxide and molecular oxygen.
Reduction: Reducing agents such as lithium aluminum hydride are often used.
Substitution: Bases like sodium hydroxide or potassium carbonate are typically employed.
Major Products:
Oxidation: Phosphonic acids.
Reduction: Phosphine derivatives.
Substitution: Various substituted phosphonates.
Mechanism of Action
The mechanism by which diphenoxyphosphanolate exerts its effects involves its interaction with molecular targets such as enzymes and receptors. It can inhibit enzyme activity by binding to the active site, thereby preventing substrate access. This interaction often involves the formation of a stable complex between the compound and the enzyme, leading to a decrease in enzymatic activity .
Comparison with Similar Compounds
- Diphenylphosphine oxide
- Phenylphosphonic acid
- Triphenylphosphine
Comparison: Diphenoxyphosphanolate is unique due to its dual phenoxy groups, which confer distinct chemical properties compared to its analogs. For instance, while diphenylphosphine oxide is primarily used as a ligand in catalysis, this compound’s phenoxy groups make it more reactive in substitution reactions .
Properties
IUPAC Name |
diphenyl phosphite | |
---|---|---|
Details | Computed by Lexichem TK 2.7.0 (PubChem release 2021.05.07) | |
Source | PubChem | |
URL | https://pubchem.ncbi.nlm.nih.gov | |
Description | Data deposited in or computed by PubChem | |
InChI |
InChI=1S/C12H10O3P/c13-16(14-11-7-3-1-4-8-11)15-12-9-5-2-6-10-12/h1-10H/q-1 | |
Details | Computed by InChI 1.0.6 (PubChem release 2021.05.07) | |
Source | PubChem | |
URL | https://pubchem.ncbi.nlm.nih.gov | |
Description | Data deposited in or computed by PubChem | |
InChI Key |
KUMNEOGIHFCNQW-UHFFFAOYSA-N | |
Details | Computed by InChI 1.0.6 (PubChem release 2021.05.07) | |
Source | PubChem | |
URL | https://pubchem.ncbi.nlm.nih.gov | |
Description | Data deposited in or computed by PubChem | |
Canonical SMILES |
C1=CC=C(C=C1)OP([O-])OC2=CC=CC=C2 | |
Details | Computed by OEChem 2.3.0 (PubChem release 2021.05.07) | |
Source | PubChem | |
URL | https://pubchem.ncbi.nlm.nih.gov | |
Description | Data deposited in or computed by PubChem | |
Molecular Formula |
C12H10O3P- | |
Details | Computed by PubChem 2.1 (PubChem release 2021.05.07) | |
Source | PubChem | |
URL | https://pubchem.ncbi.nlm.nih.gov | |
Description | Data deposited in or computed by PubChem | |
DSSTOX Substance ID |
DTXSID70633743 | |
Record name | Diphenoxyphosphanolate | |
Source | EPA DSSTox | |
URL | https://comptox.epa.gov/dashboard/DTXSID70633743 | |
Description | DSSTox provides a high quality public chemistry resource for supporting improved predictive toxicology. | |
Molecular Weight |
233.18 g/mol | |
Details | Computed by PubChem 2.1 (PubChem release 2021.05.07) | |
Source | PubChem | |
URL | https://pubchem.ncbi.nlm.nih.gov | |
Description | Data deposited in or computed by PubChem | |
CAS No. |
102-10-3 | |
Record name | Diphenoxyphosphanolate | |
Source | EPA DSSTox | |
URL | https://comptox.epa.gov/dashboard/DTXSID70633743 | |
Description | DSSTox provides a high quality public chemistry resource for supporting improved predictive toxicology. | |
Retrosynthesis Analysis
AI-Powered Synthesis Planning: Our tool employs the Template_relevance Pistachio, Template_relevance Bkms_metabolic, Template_relevance Pistachio_ringbreaker, Template_relevance Reaxys, Template_relevance Reaxys_biocatalysis model, leveraging a vast database of chemical reactions to predict feasible synthetic routes.
One-Step Synthesis Focus: Specifically designed for one-step synthesis, it provides concise and direct routes for your target compounds, streamlining the synthesis process.
Accurate Predictions: Utilizing the extensive PISTACHIO, BKMS_METABOLIC, PISTACHIO_RINGBREAKER, REAXYS, REAXYS_BIOCATALYSIS database, our tool offers high-accuracy predictions, reflecting the latest in chemical research and data.
Strategy Settings
Precursor scoring | Relevance Heuristic |
---|---|
Min. plausibility | 0.01 |
Model | Template_relevance |
Template Set | Pistachio/Bkms_metabolic/Pistachio_ringbreaker/Reaxys/Reaxys_biocatalysis |
Top-N result to add to graph | 6 |
Feasible Synthetic Routes
Disclaimer and Information on In-Vitro Research Products
Please be aware that all articles and product information presented on BenchChem are intended solely for informational purposes. The products available for purchase on BenchChem are specifically designed for in-vitro studies, which are conducted outside of living organisms. In-vitro studies, derived from the Latin term "in glass," involve experiments performed in controlled laboratory settings using cells or tissues. It is important to note that these products are not categorized as medicines or drugs, and they have not received approval from the FDA for the prevention, treatment, or cure of any medical condition, ailment, or disease. We must emphasize that any form of bodily introduction of these products into humans or animals is strictly prohibited by law. It is essential to adhere to these guidelines to ensure compliance with legal and ethical standards in research and experimentation.