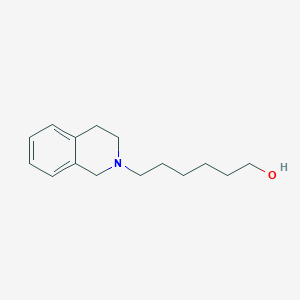
6-(3,4-Dihydroisoquinolin-2(1H)-yl)hexan-1-ol
Overview
Description
6-(3,4-Dihydroisoquinolin-2(1H)-yl)hexan-1-ol is a synthetic organic compound featuring a hexanol backbone substituted with a 3,4-dihydroisoquinoline moiety.
Preparation Methods
Synthetic Routes and Reaction Conditions
The synthesis of 6-(3,4-Dihydroisoquinolin-2(1H)-yl)hexan-1-ol typically involves the following steps:
Formation of the Isoquinoline Ring: The isoquinoline ring can be synthesized through the Bischler-Napieralski reaction, where a β-phenylethylamine derivative is cyclized using a dehydrating agent such as phosphorus oxychloride (POCl₃) or polyphosphoric acid (PPA).
Reduction to Dihydroisoquinoline: The isoquinoline ring is then reduced to dihydroisoquinoline using a reducing agent like sodium borohydride (NaBH₄) or lithium aluminum hydride (LiAlH₄).
Attachment of the Hexanol Chain: The hexanol chain can be introduced through a nucleophilic substitution reaction, where the dihydroisoquinoline is reacted with a suitable alkyl halide (e.g., 6-bromohexanol) in the presence of a base such as potassium carbonate (K₂CO₃).
Industrial Production Methods
Industrial production of this compound may involve similar synthetic routes but optimized for large-scale production. This includes the use of continuous flow reactors, automated synthesis, and efficient purification techniques such as chromatography and crystallization.
Chemical Reactions Analysis
Types of Reactions
Oxidation: The hydroxyl group in 6-(3,4-Dihydroisoquinolin-2(1H)-yl)hexan-1-ol can be oxidized to a carbonyl group using oxidizing agents like chromium trioxide (CrO₃) or pyridinium chlorochromate (PCC).
Reduction: The compound can undergo reduction reactions, particularly at the dihydroisoquinoline ring, using reducing agents such as hydrogen gas (H₂) in the presence of a palladium catalyst (Pd/C).
Substitution: The hydroxyl group can be substituted with other functional groups through reactions with reagents like thionyl chloride (SOCl₂) to form the corresponding alkyl chloride.
Common Reagents and Conditions
Oxidation: Chromium trioxide (CrO₃), pyridinium chlorochromate (PCC)
Reduction: Sodium borohydride (NaBH₄), lithium aluminum hydride (LiAlH₄), hydrogen gas (H₂) with palladium catalyst (Pd/C)
Substitution: Thionyl chloride (SOCl₂), potassium carbonate (K₂CO₃)
Major Products Formed
Oxidation: Formation of the corresponding ketone or aldehyde
Reduction: Formation of fully reduced isoquinoline derivatives
Substitution: Formation of alkyl halides or other substituted derivatives
Scientific Research Applications
Chemistry
In chemistry, 6-(3,4-Dihydroisoquinolin-2(1H)-yl)hexan-1-ol is used as an intermediate in the synthesis of more complex molecules. Its unique structure allows for the exploration of various chemical transformations and the development of new synthetic methodologies.
Biology
In biological research, this compound can be used as a probe to study the interactions of isoquinoline derivatives with biological targets. It may also serve as a starting point for the development of bioactive molecules with potential therapeutic applications.
Medicine
In medicinal chemistry, this compound is investigated for its potential pharmacological properties. It may exhibit activity against certain diseases or conditions, making it a candidate for drug development.
Industry
In the industrial sector, this compound can be utilized in the production of specialty chemicals, materials, and polymers. Its functional groups allow for modifications that can enhance the properties of industrial products.
Mechanism of Action
The mechanism of action of 6-(3,4-Dihydroisoquinolin-2(1H)-yl)hexan-1-ol depends on its specific application. In medicinal chemistry, it may interact with molecular targets such as enzymes, receptors, or ion channels. The dihydroisoquinoline ring can mimic the structure of natural ligands, allowing the compound to modulate biological pathways. The hexanol chain may also contribute to the compound’s overall activity by affecting its solubility, permeability, and binding affinity.
Comparison with Similar Compounds
Structural Analogs and Key Differences
The following compounds share the 3,4-dihydroisoquinoline scaffold but differ in substitution patterns and functional groups:
Key Observations:
- Polarity and Solubility: The hexanol group in the target compound likely enhances hydrophilicity compared to benzothiazole (4e) or amide (Compound 17) derivatives, which may exhibit lower aqueous solubility due to aromatic and nonpolar substituents .
- Pharmacological Potential: Benzothiazole derivatives (e.g., 4e) are associated with multitargeted bioactivity, including antimicrobial and anticancer effects, suggesting that the target compound may share similar therapeutic avenues .
Biological Activity
Overview
6-(3,4-Dihydroisoquinolin-2(1H)-yl)hexan-1-ol is a compound that integrates a dihydroisoquinoline moiety with a hexanol chain, making it a subject of interest in medicinal chemistry and biological research. Its unique structure allows it to interact with various biological targets, potentially leading to therapeutic applications.
- Molecular Formula : C_{15}H_{21}N
- Molecular Weight : 233.35 g/mol
- CAS Number : 88014-18-0
The biological activity of this compound is primarily attributed to its ability to mimic natural ligands, allowing it to modulate various biological pathways. The hydroxyl group enhances its solubility and binding affinity to molecular targets such as enzymes and receptors.
1. Antitumor Activity
Research indicates that derivatives of dihydroisoquinoline, including this compound, exhibit significant antitumor properties. For instance, compounds designed to inhibit Protein Arginine Methyltransferase 5 (PRMT5) have shown promising results in preclinical studies:
Compound | IC50 (nM) | GI50 (nM) | Model |
---|---|---|---|
GSK-3326595 | 5.5 | - | Clinical trial |
Compound 46 | 8.5 | 18 | MV4-11 cells |
Compound 46 demonstrated potent PRMT5 inhibition and anti-proliferative activity in leukemia models, suggesting that compounds with similar structures could serve as effective therapeutic agents against cancer .
2. Antifungal Activity
Dihydroisoquinoline derivatives have also been evaluated for their antifungal properties. A study highlighted the effectiveness of various derivatives against phytopathogens:
Compound | Inhibition Rate (%) | Target Pathogen |
---|---|---|
I23 | 96.5 (at 5 mg/pot) | Pythium recalcitrans |
Hymexazol | 63.9 | Pythium recalcitrans |
The compound I23 exhibited superior antifungal activity compared to commercial standards, indicating potential for agricultural applications .
3. Antibacterial Activity
While specific data on the antibacterial effects of this compound is limited, related quinoline derivatives have demonstrated significant antibacterial properties against various strains, suggesting that this compound may share similar activities .
Case Study 1: PRMT5 Inhibition
In a targeted study on PRMT5 inhibitors, compound derivatives were synthesized and screened for their ability to inhibit PRMT5 activity in leukemia cell lines. Compound 46 was identified as a lead candidate due to its low IC50 value and demonstrated efficacy in xenograft models .
Case Study 2: Antifungal Efficacy
A series of dihydroisoquinoline derivatives were tested against multiple fungal strains. The results indicated that structural modifications significantly influenced antifungal potency, with certain substitutions enhancing activity against specific pathogens .
Q & A
Q. What are the optimal synthetic routes for 6-(3,4-Dihydroisoquinolin-2(1H)-yl)hexan-1-ol, and how can reaction conditions be tailored to improve yield?
Basic Synthesis Methodology :
The core dihydroisoquinoline moiety can be synthesized via the Pictet-Spengler reaction , where a β-arylethylamine reacts with an aldehyde under acidic conditions . Subsequent functionalization of the hexanol chain may involve nucleophilic substitution or reductive amination. For example, coupling a pre-formed dihydroisoquinoline derivative with 6-bromohexan-1-ol under basic conditions (e.g., K₂CO₃ in DMF) could yield the target compound.
Advanced Optimization :
To maximize yield, control stereochemistry, and minimize side reactions:
- Use microwave-assisted synthesis (e.g., 90°C, 30 min) to accelerate reaction kinetics and improve regioselectivity .
- Employ Pd-catalyzed cross-coupling for C–N bond formation, leveraging cesium carbonate as a base to enhance reactivity .
- Monitor intermediates via HPLC-MS to identify byproducts (e.g., over-alkylation) and adjust stoichiometry .
Q. How can structural ambiguities in this compound be resolved using spectroscopic and crystallographic techniques?
Basic Characterization :
- NMR : Assign proton environments using ¹H/¹³C NMR. The dihydroisoquinoline protons (δ 2.5–4.0 ppm) and hexanol chain protons (δ 1.2–1.8 ppm) should show distinct splitting patterns .
- FT-IR : Confirm hydroxyl (3300–3500 cm⁻¹) and aromatic C–H (3000–3100 cm⁻¹) stretches .
Advanced Analysis :
- X-ray Crystallography : Resolve stereochemical uncertainties (e.g., chair vs. boat conformation of the dihydroisoquinoline ring) by co-crystallizing with heavy atoms or using synchrotron radiation .
- DFT Calculations : Compare experimental NMR shifts with computational models (e.g., B3LYP/6-31G*) to validate tautomeric forms .
Q. What strategies mitigate toxicity risks during in vitro pharmacological studies of this compound?
Basic Safety Protocols :
- Follow OSHA guidelines: Use fume hoods, PPE (gloves, goggles), and avoid skin contact due to potential acute toxicity (Category 4) .
- Conduct AMES tests to assess mutagenicity and MTT assays for cytotoxicity thresholds .
Advanced Risk Management :
- Use metabolite profiling (LC-HRMS) to identify toxic degradation products (e.g., quinone imines) and adjust formulation buffers (e.g., ascorbic acid to prevent oxidation) .
- Implement QSAR models to predict structure-toxicity relationships and guide structural modifications .
Q. How can contradictory data on the compound’s receptor-binding affinity be reconciled?
Basic Experimental Design :
- Standardize assay conditions (pH, temperature, solvent) to minimize variability. Use radioligand binding assays (e.g., ³H-labeled competitors) for direct affinity measurements .
Advanced Contradiction Analysis :
- Perform molecular dynamics simulations to study ligand-receptor interactions (e.g., docking to σ-1 receptors) and identify allosteric binding pockets .
- Validate results across multiple cell lines (e.g., HEK293 vs. CHO) to rule out cell-specific artifacts .
Q. What computational tools are effective for modeling the pharmacokinetic properties of this compound?
Basic ADME Prediction :
- Use SwissADME to estimate logP (lipophilicity), blood-brain barrier permeability, and CYP450 interactions .
Advanced Modeling :
- Apply PBPK models (e.g., GastroPlus) incorporating in vitro data (e.g., Caco-2 permeability) to predict oral bioavailability .
- Simulate metabolic pathways with GLORYx to prioritize metabolites for synthesis and testing .
Q. How can enantiomeric impurities in synthetic batches be detected and minimized?
Basic Quality Control :
Advanced Resolution :
- Optimize asymmetric catalysis (e.g., BINAP-Ru complexes) during key synthetic steps to enhance enantiomeric excess (ee >98%) .
- Employ vibrational circular dichroism (VCD) to confirm absolute configuration .
Q. What strategies improve the compound’s stability in aqueous formulations for in vivo studies?
Basic Stabilization :
- Use lyophilization with cryoprotectants (e.g., trehalose) or store in anhydrous DMSO at -80°C .
Advanced Formulation :
Properties
IUPAC Name |
6-(3,4-dihydro-1H-isoquinolin-2-yl)hexan-1-ol | |
---|---|---|
Details | Computed by LexiChem 2.6.6 (PubChem release 2019.06.18) | |
Source | PubChem | |
URL | https://pubchem.ncbi.nlm.nih.gov | |
Description | Data deposited in or computed by PubChem | |
InChI |
InChI=1S/C15H23NO/c17-12-6-2-1-5-10-16-11-9-14-7-3-4-8-15(14)13-16/h3-4,7-8,17H,1-2,5-6,9-13H2 | |
Details | Computed by InChI 1.0.5 (PubChem release 2019.06.18) | |
Source | PubChem | |
URL | https://pubchem.ncbi.nlm.nih.gov | |
Description | Data deposited in or computed by PubChem | |
InChI Key |
JVKCMIBKSSNORG-UHFFFAOYSA-N | |
Details | Computed by InChI 1.0.5 (PubChem release 2019.06.18) | |
Source | PubChem | |
URL | https://pubchem.ncbi.nlm.nih.gov | |
Description | Data deposited in or computed by PubChem | |
Canonical SMILES |
C1CN(CC2=CC=CC=C21)CCCCCCO | |
Details | Computed by OEChem 2.1.5 (PubChem release 2019.06.18) | |
Source | PubChem | |
URL | https://pubchem.ncbi.nlm.nih.gov | |
Description | Data deposited in or computed by PubChem | |
Molecular Formula |
C15H23NO | |
Details | Computed by PubChem 2.1 (PubChem release 2019.06.18) | |
Source | PubChem | |
URL | https://pubchem.ncbi.nlm.nih.gov | |
Description | Data deposited in or computed by PubChem | |
DSSTOX Substance ID |
DTXSID70518187 | |
Record name | 6-(3,4-Dihydroisoquinolin-2(1H)-yl)hexan-1-ol | |
Source | EPA DSSTox | |
URL | https://comptox.epa.gov/dashboard/DTXSID70518187 | |
Description | DSSTox provides a high quality public chemistry resource for supporting improved predictive toxicology. | |
Molecular Weight |
233.35 g/mol | |
Details | Computed by PubChem 2.1 (PubChem release 2021.05.07) | |
Source | PubChem | |
URL | https://pubchem.ncbi.nlm.nih.gov | |
Description | Data deposited in or computed by PubChem | |
CAS No. |
88014-18-0 | |
Record name | 6-(3,4-Dihydroisoquinolin-2(1H)-yl)hexan-1-ol | |
Source | EPA DSSTox | |
URL | https://comptox.epa.gov/dashboard/DTXSID70518187 | |
Description | DSSTox provides a high quality public chemistry resource for supporting improved predictive toxicology. | |
Retrosynthesis Analysis
AI-Powered Synthesis Planning: Our tool employs the Template_relevance Pistachio, Template_relevance Bkms_metabolic, Template_relevance Pistachio_ringbreaker, Template_relevance Reaxys, Template_relevance Reaxys_biocatalysis model, leveraging a vast database of chemical reactions to predict feasible synthetic routes.
One-Step Synthesis Focus: Specifically designed for one-step synthesis, it provides concise and direct routes for your target compounds, streamlining the synthesis process.
Accurate Predictions: Utilizing the extensive PISTACHIO, BKMS_METABOLIC, PISTACHIO_RINGBREAKER, REAXYS, REAXYS_BIOCATALYSIS database, our tool offers high-accuracy predictions, reflecting the latest in chemical research and data.
Strategy Settings
Precursor scoring | Relevance Heuristic |
---|---|
Min. plausibility | 0.01 |
Model | Template_relevance |
Template Set | Pistachio/Bkms_metabolic/Pistachio_ringbreaker/Reaxys/Reaxys_biocatalysis |
Top-N result to add to graph | 6 |
Feasible Synthetic Routes
Disclaimer and Information on In-Vitro Research Products
Please be aware that all articles and product information presented on BenchChem are intended solely for informational purposes. The products available for purchase on BenchChem are specifically designed for in-vitro studies, which are conducted outside of living organisms. In-vitro studies, derived from the Latin term "in glass," involve experiments performed in controlled laboratory settings using cells or tissues. It is important to note that these products are not categorized as medicines or drugs, and they have not received approval from the FDA for the prevention, treatment, or cure of any medical condition, ailment, or disease. We must emphasize that any form of bodily introduction of these products into humans or animals is strictly prohibited by law. It is essential to adhere to these guidelines to ensure compliance with legal and ethical standards in research and experimentation.