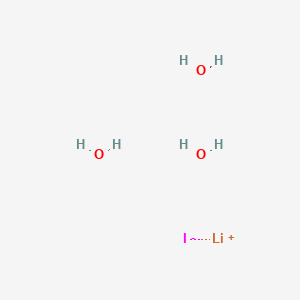
Lithium iodide trihydrate
Overview
Description
Lithium iodide trihydrate is a chemical compound composed of lithium, iodine, and water molecules. It appears as a colorless crystalline solid and is highly soluble in water and alcohol. This compound is known for its hygroscopic nature, meaning it readily absorbs moisture from the air. It is used in various applications, including as a reagent in organic synthesis and as an electrolyte in batteries.
Mechanism of Action
Target of Action
Lithium iodide trihydrate (LiI·3H2O) is a compound of lithium and iodineIt has been used in various applications due to its chemical properties .
Mode of Action
This compound interacts with its environment through its constituent ions, lithium (Li+) and iodide (I-). When exposed to air, it becomes yellow in color due to the oxidation of iodide to iodine . In organic synthesis, LiI is useful for cleaving C-O bonds .
Biochemical Pathways
Lithium iodide doesn’t directly participate in any known biochemical pathways. For example, it’s used as a solid-state electrolyte for high-temperature batteries and artificial pacemakers . It’s also used in the electrolyte of dye-sensitized solar cells .
Result of Action
The result of lithium iodide’s action depends on its application. For instance, in high-temperature batteries and artificial pacemakers, it acts as a solid-state electrolyte, enabling a long cycle life . In organic synthesis, it can cleave C-O bonds, converting methyl esters to carboxylic acids .
Action Environment
The action of lithium iodide can be influenced by environmental factors. For example, when exposed to air, it becomes yellow due to the oxidation of iodide to iodine . Its solubility varies with the solvent used, being highly soluble in water and other polar solvents .
Biochemical Analysis
Biochemical Properties
Lithium Iodide Trihydrate plays a significant role in biochemical reactions. It has been found to interact with various enzymes and proteins. For instance, in the context of lithium thiophosphate electrolytes, Lithium Iodide has been observed to play a protective role at the interface, enabling improved lithium cycling .
Molecular Mechanism
At the molecular level, this compound exerts its effects through various mechanisms. It has been observed to have binding interactions with biomolecules, potentially influencing enzyme activity and gene expression
Temporal Effects in Laboratory Settings
In laboratory settings, the effects of this compound can change over time. Factors such as the product’s stability, degradation, and long-term effects on cellular function are critical considerations in in vitro or in vivo studies .
Metabolic Pathways
This compound is involved in various metabolic pathways. It interacts with certain enzymes and cofactors, potentially influencing metabolic flux or metabolite levels
Transport and Distribution
The transport and distribution of this compound within cells and tissues involve various transporters and binding proteins
Subcellular Localization
The subcellular localization of this compound and its effects on activity or function are complex and multifaceted. Factors such as targeting signals or post-translational modifications that direct it to specific compartments or organelles could potentially influence its activity
Preparation Methods
Synthetic Routes and Reaction Conditions: Lithium iodide trihydrate can be synthesized by reacting lithium hydroxide or lithium carbonate with hydroiodic acid. The reaction typically occurs in an aqueous medium, followed by crystallization to obtain the trihydrate form. The reaction can be represented as: [ \text{2HI} + \text{Li}_2\text{CO}_3 + 3\text{H}_2\text{O} \rightarrow 2\text{LiI} \cdot 3\text{H}_2\text{O} + \text{CO}_2 ]
Industrial Production Methods: In industrial settings, this compound is produced by a similar method, but on a larger scale. The process involves careful control of reaction conditions to ensure high purity and yield. The product is then dried and packaged under inert conditions to prevent moisture absorption and degradation.
Types of Reactions:
Oxidation: Lithium iodide can undergo oxidation to form iodine.
Reduction: It can be reduced back to lithium and iodide ions.
Substitution: Lithium iodide is used in organic synthesis for nucleophilic substitution reactions, such as converting alkyl halides to alkyl iodides.
Common Reagents and Conditions:
Oxidation: Oxygen or other oxidizing agents.
Reduction: Reducing agents like lithium aluminum hydride.
Substitution: Organic halides and solvents like acetone or ethanol.
Major Products:
Oxidation: Iodine.
Reduction: Lithium and iodide ions.
Substitution: Alkyl iodides.
Scientific Research Applications
Lithium iodide trihydrate has several applications in scientific research:
Chemistry: Used as a reagent in organic synthesis, particularly for cleaving carbon-oxygen bonds.
Biology: Employed in the preparation of iodide solutions for various biological assays.
Medicine: Historically used as a radiocontrast agent in medical imaging, although its use has declined due to toxicity concerns.
Industry: Utilized as an electrolyte in high-temperature batteries and dye-sensitized solar cells.
Comparison with Similar Compounds
- Lithium fluoride
- Lithium chloride
- Lithium bromide
Comparison:
- Solubility: Lithium iodide trihydrate is more soluble in water and organic solvents compared to lithium fluoride and lithium chloride.
- Reactivity: It is more reactive in nucleophilic substitution reactions than lithium fluoride and lithium chloride.
- Applications: While lithium fluoride and lithium chloride are primarily used in different industrial applications, this compound’s unique solubility and reactivity make it particularly valuable in organic synthesis and as an electrolyte in batteries.
This compound stands out due to its high solubility, reactivity, and versatility in various scientific and industrial applications. Its ability to participate in multiple types of chemical reactions and its role in advanced technologies highlight its importance in modern chemistry and industry.
Properties
IUPAC Name |
lithium;iodide;trihydrate | |
---|---|---|
Details | Computed by Lexichem TK 2.7.0 (PubChem release 2021.05.07) | |
Source | PubChem | |
URL | https://pubchem.ncbi.nlm.nih.gov | |
Description | Data deposited in or computed by PubChem | |
InChI |
InChI=1S/HI.Li.3H2O/h1H;;3*1H2/q;+1;;;/p-1 | |
Details | Computed by InChI 1.0.6 (PubChem release 2021.05.07) | |
Source | PubChem | |
URL | https://pubchem.ncbi.nlm.nih.gov | |
Description | Data deposited in or computed by PubChem | |
InChI Key |
UMXWTWTZJKLUKQ-UHFFFAOYSA-M | |
Details | Computed by InChI 1.0.6 (PubChem release 2021.05.07) | |
Source | PubChem | |
URL | https://pubchem.ncbi.nlm.nih.gov | |
Description | Data deposited in or computed by PubChem | |
Canonical SMILES |
[Li+].O.O.O.[I-] | |
Details | Computed by OEChem 2.3.0 (PubChem release 2021.05.07) | |
Source | PubChem | |
URL | https://pubchem.ncbi.nlm.nih.gov | |
Description | Data deposited in or computed by PubChem | |
Molecular Formula |
H6ILiO3 | |
Details | Computed by PubChem 2.1 (PubChem release 2021.05.07) | |
Source | PubChem | |
URL | https://pubchem.ncbi.nlm.nih.gov | |
Description | Data deposited in or computed by PubChem | |
DSSTOX Substance ID |
DTXSID60635630 | |
Record name | Lithium iodide--water (1/1/3) | |
Source | EPA DSSTox | |
URL | https://comptox.epa.gov/dashboard/DTXSID60635630 | |
Description | DSSTox provides a high quality public chemistry resource for supporting improved predictive toxicology. | |
Molecular Weight |
187.9 g/mol | |
Details | Computed by PubChem 2.1 (PubChem release 2021.05.07) | |
Source | PubChem | |
URL | https://pubchem.ncbi.nlm.nih.gov | |
Description | Data deposited in or computed by PubChem | |
CAS No. |
7790-22-9 | |
Record name | Lithium iodide trihydrate | |
Source | ChemIDplus | |
URL | https://pubchem.ncbi.nlm.nih.gov/substance/?source=chemidplus&sourceid=0007790229 | |
Description | ChemIDplus is a free, web search system that provides access to the structure and nomenclature authority files used for the identification of chemical substances cited in National Library of Medicine (NLM) databases, including the TOXNET system. | |
Record name | Lithium iodide--water (1/1/3) | |
Source | EPA DSSTox | |
URL | https://comptox.epa.gov/dashboard/DTXSID60635630 | |
Description | DSSTox provides a high quality public chemistry resource for supporting improved predictive toxicology. | |
Record name | LITHIUM IODIDE TRIHYDRATE | |
Source | FDA Global Substance Registration System (GSRS) | |
URL | https://gsrs.ncats.nih.gov/ginas/app/beta/substances/PS8215OJNR | |
Description | The FDA Global Substance Registration System (GSRS) enables the efficient and accurate exchange of information on what substances are in regulated products. Instead of relying on names, which vary across regulatory domains, countries, and regions, the GSRS knowledge base makes it possible for substances to be defined by standardized, scientific descriptions. | |
Explanation | Unless otherwise noted, the contents of the FDA website (www.fda.gov), both text and graphics, are not copyrighted. They are in the public domain and may be republished, reprinted and otherwise used freely by anyone without the need to obtain permission from FDA. Credit to the U.S. Food and Drug Administration as the source is appreciated but not required. | |
Retrosynthesis Analysis
AI-Powered Synthesis Planning: Our tool employs the Template_relevance Pistachio, Template_relevance Bkms_metabolic, Template_relevance Pistachio_ringbreaker, Template_relevance Reaxys, Template_relevance Reaxys_biocatalysis model, leveraging a vast database of chemical reactions to predict feasible synthetic routes.
One-Step Synthesis Focus: Specifically designed for one-step synthesis, it provides concise and direct routes for your target compounds, streamlining the synthesis process.
Accurate Predictions: Utilizing the extensive PISTACHIO, BKMS_METABOLIC, PISTACHIO_RINGBREAKER, REAXYS, REAXYS_BIOCATALYSIS database, our tool offers high-accuracy predictions, reflecting the latest in chemical research and data.
Strategy Settings
Precursor scoring | Relevance Heuristic |
---|---|
Min. plausibility | 0.01 |
Model | Template_relevance |
Template Set | Pistachio/Bkms_metabolic/Pistachio_ringbreaker/Reaxys/Reaxys_biocatalysis |
Top-N result to add to graph | 6 |
Feasible Synthetic Routes
Q1: What are the main applications of lithium iodide trihydrate?
A1: this compound serves as a precursor for producing high-purity anhydrous lithium iodide, a crucial component in various electrochemical applications like batteries. Additionally, it plays a role in synthesizing specific organic compounds. For instance, it facilitates the high-yield reduction of 2-O-trifluoromethanesulfonate esters of α-hydroxylactones to their corresponding 2-deoxylactones [, ].
Q2: How does the presence of silica impact the conductivity of this compound?
A2: Studies have demonstrated that incorporating silica into this compound can significantly enhance its ionic conductivity. This effect is observed in materials with compositions near both the trihydrate and monohydrate forms. The conductivity increase is attributed to the formation of dispersed-phase solids within the LiI-H2O-SiO2 system [].
Q3: Are there alternative materials to this compound in its applications?
A3: While this compound serves as a precursor to anhydrous lithium iodide, which is valuable in battery technologies, exploring alternative lithium salts for similar applications is an active research area. These alternatives could offer advantages in terms of cost, performance, or environmental impact [].
Q4: What are the challenges in preparing high-purity anhydrous lithium iodide from this compound?
A5: One challenge lies in achieving a product with extremely low water content, as required for battery-grade anhydrous lithium iodide. Methods often involve multiple drying steps, including pre-drying and vacuum drying, to meet these stringent purity standards [].
Q5: How is lithium sulfate monohydrate waste generated during this compound production utilized?
A6: Interestingly, lithium sulfate monohydrate, a byproduct of this compound synthesis, can be repurposed to produce high-purity anhydrous lithium sulfate. This approach offers a sustainable solution for waste management and reduces reliance on virgin materials [].
Q6: Have there been theoretical studies on the crystallization of this compound?
A7: Yes, statistical mechanical theories combined with molecular modeling have been employed to understand the crystallization of this compound from aqueous solutions. These studies provide insights into the thermodynamic factors governing its formation and stability [].
Disclaimer and Information on In-Vitro Research Products
Please be aware that all articles and product information presented on BenchChem are intended solely for informational purposes. The products available for purchase on BenchChem are specifically designed for in-vitro studies, which are conducted outside of living organisms. In-vitro studies, derived from the Latin term "in glass," involve experiments performed in controlled laboratory settings using cells or tissues. It is important to note that these products are not categorized as medicines or drugs, and they have not received approval from the FDA for the prevention, treatment, or cure of any medical condition, ailment, or disease. We must emphasize that any form of bodily introduction of these products into humans or animals is strictly prohibited by law. It is essential to adhere to these guidelines to ensure compliance with legal and ethical standards in research and experimentation.