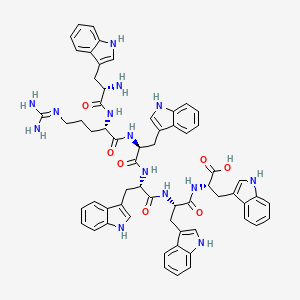
Trp-Arg-Trp-Trp-Trp-Trp
Overview
Description
Trp-Arg-Trp-Trp-Trp-Trp (WRW4) is a synthetic hexapeptide characterized by a tryptophan (Trp)-rich sequence with a single arginine (Arg) residue. It is widely recognized as a potent antagonist of formyl peptide receptor-like 2 (FPR2/3), a G-protein-coupled receptor involved in inflammatory responses, immune regulation, and disease pathogenesis . WRW4 was first identified by Shin et al. (2006), who demonstrated its ability to block FPR2-mediated signaling pathways, including calcium mobilization and reactive oxygen species (ROS) production in neutrophils . Subsequent studies have expanded its applications to cellular models of insulin resistance, Alzheimer’s disease, and wound healing, highlighting its versatility in modulating receptor-specific pathways .
Preparation Methods
Synthetic Routes and Reaction Conditions
WRW4-OH (trifluoroacetate salt) is synthesized through solid-phase peptide synthesis (SPPS). This method involves the sequential addition of amino acids to a growing peptide chain anchored to a solid resin. The process typically includes the following steps:
Resin Loading: The first amino acid is attached to the resin.
Deprotection: The protecting group on the amino acid is removed.
Coupling: The next amino acid is activated and coupled to the growing peptide chain.
Repetition: Steps 2 and 3 are repeated until the desired peptide sequence is obtained.
Cleavage and Purification: The peptide is cleaved from the resin and purified.
Industrial Production Methods
Industrial production of WRW4-OH (trifluoroacetate salt) follows similar principles as laboratory synthesis but on a larger scale. Automated peptide synthesizers and advanced purification techniques such as high-performance liquid chromatography (HPLC) are employed to ensure high purity and yield .
Chemical Reactions Analysis
Types of Reactions
WRW4-OH (trifluoroacetate salt) primarily undergoes peptide bond formation and hydrolysis reactions. It does not typically participate in oxidation, reduction, or substitution reactions due to its peptide nature .
Common Reagents and Conditions
Peptide Bond Formation: Reagents such as N,N’-diisopropylcarbodiimide (DIC) and hydroxybenzotriazole (HOBt) are commonly used.
Hydrolysis: Acidic or basic conditions can induce hydrolysis of peptide bonds.
Major Products
The major products formed from the reactions involving WRW4-OH (trifluoroacetate salt) are typically shorter peptide fragments resulting from hydrolysis .
Scientific Research Applications
Antimicrobial Applications
Antimicrobial Peptides (AMPs)
Trp-Arg-rich peptides are recognized for their potent antimicrobial properties. Research indicates that these peptides can effectively disrupt bacterial membranes, making them promising candidates for overcoming antibiotic resistance. A study highlighted the development of a library of Trp- and Arg-rich engineered peptides tested against multidrug-resistant bacteria known as ESKAPE pathogens. The results showed that these peptides had minimum inhibitory concentrations (MIC) ranging from 2–4 μM with negligible toxicity to mammalian cells .
Mechanism of Action
The mechanism by which Trp-Arg peptides exert their antimicrobial effects involves electrostatic interactions with bacterial membranes, facilitated by the positively charged Arg residues. Modifications to the peptide structure, such as substituting Arg with Lys, have been explored to enhance stability and activity against bacterial strains. It was found that while Arg residues promote membrane permeabilization, Lys substitutions tend to reduce antimicrobial potency .
Agricultural Biotechnology
Trp Accumulation in Crops
In agricultural research, Trp-Arg peptides have been utilized to enhance the nutritional profile of crops. A notable study demonstrated the successful production of Trp-accumulating rice through gene targeting (GT) strategies. The homozygous GT plants exhibited Trp levels up to 230-fold higher than non-transformants without morphological changes . This bioengineering approach not only addresses nutritional deficiencies but also enhances the feed quality for livestock.
Nutritional Benefits
Tryptophan is an essential amino acid crucial for human health and livestock nutrition, contributing to serotonin production and other vital metabolites. Increasing Trp levels in crops could help combat deficiencies in diets globally, especially in regions reliant on cereal-based foods .
Therapeutic Potential
Receptor Agonism
There is emerging evidence suggesting that Trp-Arg peptides may act as agonists for various receptors, including the β2 adrenergic receptor (β2AR). A study identified a unique tripeptide, Arg-His-Trp, derived from serum that showed potential agonistic activity towards β2AR, which is relevant in treating conditions such as asthma and chronic obstructive pulmonary disease (COPD) . Although this specific peptide differs from Trp-Arg-Trp-Trp-Trp-Trp, it underscores the therapeutic potential of similar sequences.
Structural Stability Studies
Research into the structural properties of Trp-rich peptides has shown that they can form stable secondary structures, such as α-helices and β-hairpins, which are critical for their biological function. The stability of these structures can be influenced by environmental factors such as organic salts . Understanding these interactions is crucial for designing effective therapeutic peptides.
Summary Table of Applications
Mechanism of Action
WRW4-OH (trifluoroacetate salt) exerts its effects by binding to and antagonizing formyl peptide receptor 2 (FPR2). This interaction inhibits the receptor’s ability to mediate intracellular signaling pathways, such as calcium mobilization and chemotaxis. The compound’s mechanism of action involves blocking the binding of agonists to FPR2, thereby preventing downstream signaling events .
Comparison with Similar Compounds
Structural Analogues: Trp/Arg-Rich Hexapeptides
WRW4 belongs to a class of Trp/Arg-rich peptides, which exhibit diverse biological activities depending on their sequence and residue composition. Key analogues include:
Key Differences :
- Residue Position 5 : The polarity of the fifth residue (Cys/Thr vs. Phe) critically influences biological efficacy. Polar residues enhance wound healing by promoting cell migration and vascularization .
- Receptor Specificity : Unlike WRW4, which targets FPR2/3, RRWWCR/RRWWTR act via undefined receptors in wound repair pathways.
FPR2 Ligands: Agonists vs. Antagonists
WRW4 is distinct from other FPR2 ligands in its antagonistic activity. Comparative analysis with FPR2 agonists and antagonists:
Key Differences :
- Mechanism : WRW4 and pyrazolon derivatives antagonize FPR2, but WRW4’s peptide structure allows for higher receptor specificity compared to small molecules .
- Therapeutic Scope : WRW4’s dual role in inflammation and neurodegeneration contrasts with F2L’s anti-angiogenic focus .
Non-Peptide FPR Antagonists
WRW4 is contrasted with non-peptide antagonists in terms of bioavailability and stability:
Key Insight : While small molecules offer better pharmacokinetics, WRW4’s peptide backbone provides precise modulation of FPR2/3, making it invaluable in mechanistic studies .
Spectroscopic and Functional Comparisons
highlights structural differences between WRW4 and other peptides using surface-enhanced Raman spectroscopy (SERS):
Biological Activity
Trp-Arg-Trp-Trp-Trp-Trp (commonly referred to as WRW4) is a synthetic peptide composed of a repetitive sequence of tryptophan (Trp) and arginine (Arg) residues. This peptide has garnered attention in biomedical research due to its diverse biological activities, including antioxidant properties, receptor antagonism, and potential therapeutic applications.
Chemical Structure
The structure of WRW4 can be represented as follows:
- Sequence : this compound
- Molecular Formula : C₃₁H₄₃N₉O₆S
- Molecular Weight : 651.80 g/mol
The peptide features an amide group at the C-terminus, enhancing its stability and biological activity.
1. Antioxidant Properties
Research indicates that WRW4 exhibits significant antioxidant activity. A study comparing various tripeptides found that peptides containing Trp residues demonstrated superior radical scavenging capabilities. The antioxidant activity was measured using assays such as ABTS and ORAC, revealing that Trp-containing peptides effectively scavenge free radicals, thus potentially mitigating oxidative stress in biological systems .
2. Receptor Antagonism
WRW4 has been identified as an antagonist of the Formyl Peptide Receptor 2 (FPR2), which plays a crucial role in inflammatory responses. In vitro studies showed that WRW4 could inhibit neutrophil activation, suggesting its utility in managing inflammatory conditions . The compound has been used in experimental models to visualize inflammation and assess its therapeutic efficacy.
3. Antimicrobial Activity
Recent studies have explored the antimicrobial properties of WRW4 and similar peptides. It has been shown that Trp-rich peptides can disrupt bacterial membranes, making them effective against multidrug-resistant strains. The minimum inhibitory concentration (MIC) for some engineered peptides in this class was reported to be between 2–4 μM, indicating promising antibacterial potential .
Case Study 1: Inflammation Imaging
In a study aimed at visualizing acute inflammation, WRW4 was utilized as a fluorescent probe targeting neutrophils. The results demonstrated its effectiveness in distinguishing between activated and non-activated states of neutrophils, providing insights into inflammatory processes .
Case Study 2: Antimicrobial Testing
A series of engineered peptides based on the Trp-Arg framework were tested against ESKAPE pathogens, known for their antibiotic resistance. The findings revealed that specific modifications to the Trp residues significantly enhanced antimicrobial activity while maintaining low toxicity to mammalian cells .
Research Findings Summary Table
Biological Activity | Description | Assay/Method | Key Findings |
---|---|---|---|
Antioxidant Activity | Scavenging of free radicals | ABTS, ORAC assays | High radical scavenging capacity due to Trp residues |
Receptor Antagonism | Inhibition of FPR2 activation | In vitro neutrophil assays | Effective in reducing neutrophil activation |
Antimicrobial Activity | Disruption of bacterial membranes | MIC testing against ESKAPE pathogens | MIC values between 2–4 μM with low mammalian toxicity |
Q & A
Basic Research Questions
Q. What are the primary structural characteristics of Trp-Arg-Trp-Trp-Trp-Trp, and how do they influence its biological activity?
- Methodological Answer : The peptide's structure is dominated by its repetitive tryptophan (Trp) residues and a central arginine (Arg), which confer amphipathic properties. Techniques such as circular dichroism (CD) spectroscopy and nuclear magnetic resonance (NMR) are critical for analyzing secondary structures (e.g., β-sheet or α-helix propensity). Surface-enhanced Raman spectroscopy (SERS) on gold colloids has been used to study its vibrational modes, particularly Trp side-chain interactions with metallic surfaces . Molecular dynamics simulations can further elucidate how structural flexibility affects receptor binding (e.g., Fpr2/3 activation) .
Q. Which experimental models are suitable for studying the anti-inflammatory effects of this compound?
- Methodological Answer : In vitro models like lipopolysaccharide (LPS)-stimulated macrophages are widely used to assess cytokine suppression (e.g., TNFα, IL-10). For receptor-specific studies, Fpr2/3-transfected cell lines enable targeted analysis of ligand-receptor interactions. In vivo murine models of acute inflammation (e.g., peritonitis) can validate therapeutic potential, with dose-response experiments and knockout controls to confirm mechanism specificity .
Q. How can researchers optimize the stability of this compound in physiological conditions?
- Methodological Answer : Stability assays under varying pH, temperature, and protease exposure are essential. High-performance liquid chromatography (HPLC) with mass spectrometry (MS) monitors degradation products. Peptide engineering strategies, such as D-amino acid substitution or cyclization, can enhance resistance to enzymatic cleavage. Circular dichroism (CD) under simulated physiological buffers (e.g., PBS) provides insights into structural resilience .
Advanced Research Questions
Q. What are the challenges in reconciling contradictory data on this compound's binding affinity across different receptor isoforms?
- Methodological Answer : Discrepancies may arise from isoform-specific post-translational modifications or assay conditions (e.g., buffer ionic strength). Comparative studies using isothermal titration calorimetry (ITC) and surface plasmon resonance (SPR) can quantify binding kinetics under standardized conditions. Computational docking simulations with receptor homology models help identify isoform-specific binding pockets, guiding mutagenesis experiments to validate critical residues .
Q. How can multi-parameter optimization frameworks improve the design of this compound derivatives for enhanced bioactivity?
- Methodological Answer : Machine learning-driven QSAR (quantitative structure-activity relationship) models integrate parameters like hydrophobicity, charge distribution, and steric bulk. High-throughput synthesis coupled with functional screens (e.g., NFκB inhibition assays) identifies lead candidates. Free-energy perturbation (FEP) calculations predict binding energy changes for specific substitutions, prioritizing synthetic targets .
Q. What strategies mitigate interference from Trp fluorescence in spectroscopic studies of this compound?
- Methodological Answer : Time-resolved fluorescence or Förster resonance energy transfer (FRET) with non-Trp quenchers (e.g., acrylamide) reduces autofluorescence artifacts. SERS with silver island films shifts the Raman signal to regions less affected by Trp emission. Deuterated solvents or low-temperature measurements can further suppress background noise .
Q. Data Interpretation and Reporting
Q. How should researchers address variability in SERS spectra of this compound due to substrate heterogeneity?
- Methodological Answer : Normalize spectra using internal standards (e.g., adenine peaks) and aggregate data from ≥3 substrate batches. Principal component analysis (PCA) distinguishes peptide-specific signals from substrate noise. Atomic force microscopy (AFM) correlates spectral features with nanoparticle morphology .
Q. What statistical approaches are recommended for analyzing dose-dependent cytokine modulation by this compound?
- Methodological Answer : Nonlinear regression (e.g., sigmoidal dose-response curves) calculates EC50/IC50 values. Mixed-effects models account for inter-experiment variability. Bootstrap resampling validates confidence intervals, especially with small sample sizes. False discovery rate (FDR) correction is critical for multi-cytokine panels .
Q. Tables for Key Experimental Parameters
Q. Guidelines for Reporting
- Abstracts : Include structural insights, receptor specificity, and translational implications. Use keywords like "anti-inflammatory peptide," "Fpr2/3 agonist," and "SERS spectroscopy" for searchability .
- Data Contradictions : Explicitly discuss assay limitations (e.g., buffer composition, cell type) in the Methods section. Use supplemental figures to compare conflicting datasets .
Properties
IUPAC Name |
(2S)-2-[[(2S)-2-[[(2S)-2-[[(2S)-2-[[(2S)-2-[[(2S)-2-amino-3-(1H-indol-3-yl)propanoyl]amino]-5-(diaminomethylideneamino)pentanoyl]amino]-3-(1H-indol-3-yl)propanoyl]amino]-3-(1H-indol-3-yl)propanoyl]amino]-3-(1H-indol-3-yl)propanoyl]amino]-3-(1H-indol-3-yl)propanoic acid | |
---|---|---|
Details | Computed by Lexichem TK 2.7.0 (PubChem release 2021.05.07) | |
Source | PubChem | |
URL | https://pubchem.ncbi.nlm.nih.gov | |
Description | Data deposited in or computed by PubChem | |
InChI |
InChI=1S/C61H64N14O7/c62-44(24-34-29-66-45-17-6-1-12-39(34)45)55(76)71-50(22-11-23-65-61(63)64)56(77)72-51(25-35-30-67-46-18-7-2-13-40(35)46)57(78)73-52(26-36-31-68-47-19-8-3-14-41(36)47)58(79)74-53(27-37-32-69-48-20-9-4-15-42(37)48)59(80)75-54(60(81)82)28-38-33-70-49-21-10-5-16-43(38)49/h1-10,12-21,29-33,44,50-54,66-70H,11,22-28,62H2,(H,71,76)(H,72,77)(H,73,78)(H,74,79)(H,75,80)(H,81,82)(H4,63,64,65)/t44-,50-,51-,52-,53-,54-/m0/s1 | |
Details | Computed by InChI 1.0.6 (PubChem release 2021.05.07) | |
Source | PubChem | |
URL | https://pubchem.ncbi.nlm.nih.gov | |
Description | Data deposited in or computed by PubChem | |
InChI Key |
VVXWFNAZHNGTHK-WOAIKHIASA-N | |
Details | Computed by InChI 1.0.6 (PubChem release 2021.05.07) | |
Source | PubChem | |
URL | https://pubchem.ncbi.nlm.nih.gov | |
Description | Data deposited in or computed by PubChem | |
Canonical SMILES |
C1=CC=C2C(=C1)C(=CN2)CC(C(=O)NC(CCCN=C(N)N)C(=O)NC(CC3=CNC4=CC=CC=C43)C(=O)NC(CC5=CNC6=CC=CC=C65)C(=O)NC(CC7=CNC8=CC=CC=C87)C(=O)NC(CC9=CNC1=CC=CC=C19)C(=O)O)N | |
Details | Computed by OEChem 2.3.0 (PubChem release 2021.05.07) | |
Source | PubChem | |
URL | https://pubchem.ncbi.nlm.nih.gov | |
Description | Data deposited in or computed by PubChem | |
Isomeric SMILES |
C1=CC=C2C(=C1)C(=CN2)C[C@@H](C(=O)N[C@@H](CCCN=C(N)N)C(=O)N[C@@H](CC3=CNC4=CC=CC=C43)C(=O)N[C@@H](CC5=CNC6=CC=CC=C65)C(=O)N[C@@H](CC7=CNC8=CC=CC=C87)C(=O)N[C@@H](CC9=CNC1=CC=CC=C19)C(=O)O)N | |
Details | Computed by OEChem 2.3.0 (PubChem release 2021.05.07) | |
Source | PubChem | |
URL | https://pubchem.ncbi.nlm.nih.gov | |
Description | Data deposited in or computed by PubChem | |
Molecular Formula |
C61H64N14O7 | |
Details | Computed by PubChem 2.1 (PubChem release 2021.05.07) | |
Source | PubChem | |
URL | https://pubchem.ncbi.nlm.nih.gov | |
Description | Data deposited in or computed by PubChem | |
DSSTOX Substance ID |
DTXSID70648690 | |
Record name | L-Tryptophyl-N~5~-(diaminomethylidene)-L-ornithyl-L-tryptophyl-L-tryptophyl-L-tryptophyl-L-tryptophan | |
Source | EPA DSSTox | |
URL | https://comptox.epa.gov/dashboard/DTXSID70648690 | |
Description | DSSTox provides a high quality public chemistry resource for supporting improved predictive toxicology. | |
Molecular Weight |
1105.2 g/mol | |
Details | Computed by PubChem 2.1 (PubChem release 2021.05.07) | |
Source | PubChem | |
URL | https://pubchem.ncbi.nlm.nih.gov | |
Description | Data deposited in or computed by PubChem | |
CAS No. |
722541-91-5 | |
Record name | L-Tryptophyl-N~5~-(diaminomethylidene)-L-ornithyl-L-tryptophyl-L-tryptophyl-L-tryptophyl-L-tryptophan | |
Source | EPA DSSTox | |
URL | https://comptox.epa.gov/dashboard/DTXSID70648690 | |
Description | DSSTox provides a high quality public chemistry resource for supporting improved predictive toxicology. | |
Retrosynthesis Analysis
AI-Powered Synthesis Planning: Our tool employs the Template_relevance Pistachio, Template_relevance Bkms_metabolic, Template_relevance Pistachio_ringbreaker, Template_relevance Reaxys, Template_relevance Reaxys_biocatalysis model, leveraging a vast database of chemical reactions to predict feasible synthetic routes.
One-Step Synthesis Focus: Specifically designed for one-step synthesis, it provides concise and direct routes for your target compounds, streamlining the synthesis process.
Accurate Predictions: Utilizing the extensive PISTACHIO, BKMS_METABOLIC, PISTACHIO_RINGBREAKER, REAXYS, REAXYS_BIOCATALYSIS database, our tool offers high-accuracy predictions, reflecting the latest in chemical research and data.
Strategy Settings
Precursor scoring | Relevance Heuristic |
---|---|
Min. plausibility | 0.01 |
Model | Template_relevance |
Template Set | Pistachio/Bkms_metabolic/Pistachio_ringbreaker/Reaxys/Reaxys_biocatalysis |
Top-N result to add to graph | 6 |
Feasible Synthetic Routes
Disclaimer and Information on In-Vitro Research Products
Please be aware that all articles and product information presented on BenchChem are intended solely for informational purposes. The products available for purchase on BenchChem are specifically designed for in-vitro studies, which are conducted outside of living organisms. In-vitro studies, derived from the Latin term "in glass," involve experiments performed in controlled laboratory settings using cells or tissues. It is important to note that these products are not categorized as medicines or drugs, and they have not received approval from the FDA for the prevention, treatment, or cure of any medical condition, ailment, or disease. We must emphasize that any form of bodily introduction of these products into humans or animals is strictly prohibited by law. It is essential to adhere to these guidelines to ensure compliance with legal and ethical standards in research and experimentation.