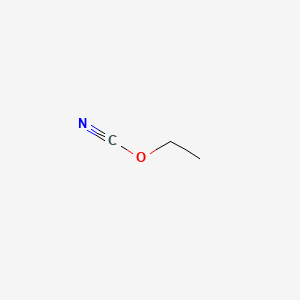
Ethyl cyanate
Overview
Description
Ethyl cyanate is an organic compound with the chemical formula C₃H₅NO. It belongs to the family of cyanates, which are esters of cyanic acid. This compound is known for its reactivity and versatility in organic synthesis, making it a valuable compound in various chemical processes.
Preparation Methods
Synthetic Routes and Reaction Conditions: Ethyl cyanate can be synthesized through several methods. One common approach involves the thermolysis of a thiatriazole intermediate. This method is efficient and widely used in laboratory settings . Another method involves the reaction of ethyl chloroformate with sodium cyanate under controlled conditions .
Industrial Production Methods: In industrial settings, this compound is typically produced by the reaction of ethyl alcohol with cyanogen chloride in the presence of a base. This method ensures high yield and purity of the product .
Chemical Reactions Analysis
Types of Reactions: Ethyl cyanate undergoes various chemical reactions, including:
Nucleophilic Substitution: this compound reacts with nucleophiles such as amines and alcohols to form urethanes and carbamates.
Hydrolysis: In the presence of water, this compound hydrolyzes to form ethyl alcohol and cyanic acid.
Oxidation and Reduction: this compound can be oxidized to form corresponding nitriles and reduced to form amines.
Common Reagents and Conditions:
Nucleophiles: Amines, alcohols
Oxidizing Agents: Peroxides, permanganates
Reducing Agents: Hydrogen, metal hydrides
Major Products Formed:
Urethanes and Carbamates: Formed through nucleophilic substitution
Nitriles and Amines: Formed through oxidation and reduction reactions
Scientific Research Applications
Ethyl cyanate has a wide range of applications in scientific research:
Chemistry: Used as a reagent in organic synthesis for the preparation of various compounds.
Biology: Employed in the synthesis of biologically active molecules and pharmaceuticals.
Medicine: Utilized in the development of drugs and therapeutic agents.
Industry: Applied in the production of polymers, resins, and other industrial chemicals.
Mechanism of Action
The mechanism of action of ethyl cyanate involves its reactivity with nucleophiles. The cyanate group (−O−C≡N) acts as an electrophile, making it susceptible to nucleophilic attack. This leads to the formation of various products depending on the nature of the nucleophile and reaction conditions . The molecular targets and pathways involved include the formation of urethanes, carbamates, and other derivatives through nucleophilic substitution reactions .
Comparison with Similar Compounds
- Methyl cyanate
- Phenyl cyanate
- Isocyanates (e.g., ethyl isocyanate)
Properties
IUPAC Name |
ethyl cyanate | |
---|---|---|
Details | Computed by Lexichem TK 2.7.0 (PubChem release 2021.05.07) | |
Source | PubChem | |
URL | https://pubchem.ncbi.nlm.nih.gov | |
Description | Data deposited in or computed by PubChem | |
InChI |
InChI=1S/C3H5NO/c1-2-5-3-4/h2H2,1H3 | |
Details | Computed by InChI 1.0.6 (PubChem release 2021.05.07) | |
Source | PubChem | |
URL | https://pubchem.ncbi.nlm.nih.gov | |
Description | Data deposited in or computed by PubChem | |
InChI Key |
JXBPSENIJJPTCI-UHFFFAOYSA-N | |
Details | Computed by InChI 1.0.6 (PubChem release 2021.05.07) | |
Source | PubChem | |
URL | https://pubchem.ncbi.nlm.nih.gov | |
Description | Data deposited in or computed by PubChem | |
Canonical SMILES |
CCOC#N | |
Details | Computed by OEChem 2.3.0 (PubChem release 2021.05.07) | |
Source | PubChem | |
URL | https://pubchem.ncbi.nlm.nih.gov | |
Description | Data deposited in or computed by PubChem | |
Molecular Formula |
C3H5NO | |
Details | Computed by PubChem 2.1 (PubChem release 2021.05.07) | |
Source | PubChem | |
URL | https://pubchem.ncbi.nlm.nih.gov | |
Description | Data deposited in or computed by PubChem | |
DSSTOX Substance ID |
DTXSID70336424 | |
Record name | Ethyl cyanate | |
Source | EPA DSSTox | |
URL | https://comptox.epa.gov/dashboard/DTXSID70336424 | |
Description | DSSTox provides a high quality public chemistry resource for supporting improved predictive toxicology. | |
Molecular Weight |
71.08 g/mol | |
Details | Computed by PubChem 2.1 (PubChem release 2021.05.07) | |
Source | PubChem | |
URL | https://pubchem.ncbi.nlm.nih.gov | |
Description | Data deposited in or computed by PubChem | |
CAS No. |
627-48-5 | |
Record name | Cyanic acid, ethyl ester | |
Source | CAS Common Chemistry | |
URL | https://commonchemistry.cas.org/detail?cas_rn=627-48-5 | |
Description | CAS Common Chemistry is an open community resource for accessing chemical information. Nearly 500,000 chemical substances from CAS REGISTRY cover areas of community interest, including common and frequently regulated chemicals, and those relevant to high school and undergraduate chemistry classes. This chemical information, curated by our expert scientists, is provided in alignment with our mission as a division of the American Chemical Society. | |
Explanation | The data from CAS Common Chemistry is provided under a CC-BY-NC 4.0 license, unless otherwise stated. | |
Record name | Ethyl cyanate | |
Source | EPA DSSTox | |
URL | https://comptox.epa.gov/dashboard/DTXSID70336424 | |
Description | DSSTox provides a high quality public chemistry resource for supporting improved predictive toxicology. | |
Retrosynthesis Analysis
AI-Powered Synthesis Planning: Our tool employs the Template_relevance Pistachio, Template_relevance Bkms_metabolic, Template_relevance Pistachio_ringbreaker, Template_relevance Reaxys, Template_relevance Reaxys_biocatalysis model, leveraging a vast database of chemical reactions to predict feasible synthetic routes.
One-Step Synthesis Focus: Specifically designed for one-step synthesis, it provides concise and direct routes for your target compounds, streamlining the synthesis process.
Accurate Predictions: Utilizing the extensive PISTACHIO, BKMS_METABOLIC, PISTACHIO_RINGBREAKER, REAXYS, REAXYS_BIOCATALYSIS database, our tool offers high-accuracy predictions, reflecting the latest in chemical research and data.
Strategy Settings
Precursor scoring | Relevance Heuristic |
---|---|
Min. plausibility | 0.01 |
Model | Template_relevance |
Template Set | Pistachio/Bkms_metabolic/Pistachio_ringbreaker/Reaxys/Reaxys_biocatalysis |
Top-N result to add to graph | 6 |
Feasible Synthetic Routes
Disclaimer and Information on In-Vitro Research Products
Please be aware that all articles and product information presented on BenchChem are intended solely for informational purposes. The products available for purchase on BenchChem are specifically designed for in-vitro studies, which are conducted outside of living organisms. In-vitro studies, derived from the Latin term "in glass," involve experiments performed in controlled laboratory settings using cells or tissues. It is important to note that these products are not categorized as medicines or drugs, and they have not received approval from the FDA for the prevention, treatment, or cure of any medical condition, ailment, or disease. We must emphasize that any form of bodily introduction of these products into humans or animals is strictly prohibited by law. It is essential to adhere to these guidelines to ensure compliance with legal and ethical standards in research and experimentation.