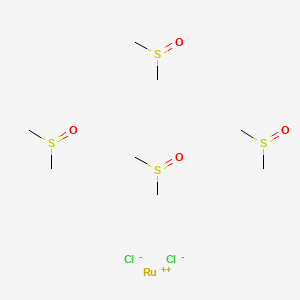
Cis-ruthenium Chloride
- Click on QUICK INQUIRY to receive a quote from our team of experts.
- With the quality product at a COMPETITIVE price, you can focus more on your research.
Overview
Description
Mechanism of Action
Target of Action
Cis-Ruthenium Chloride primarily targets human lung carcinoma tumor cell line A549 . It also presents a cytotoxic activity towards normal human peripheral blood mononuclear cells (PBMC), but only at very high concentrations .
Mode of Action
This compound interacts with its targets, leading to a reduction in the viability of A549 cells when treated with specific concentrations of the compound . It also presents a dual role on PBMC, stimulating proliferation and interleukin-2 (IL-2) production at low concentration and inducing cytotoxicity, inability to proliferate, and inhibiting IL-2 production at high concentration .
Biochemical Pathways
The compound leads to an accumulation of A549 cells in S phase and an increase in the sub-G1 peak . It induces apoptosis, as observed by the increased numbers of annexin V-positive cells and increased messenger RNA expression of caspase-3 .
Pharmacokinetics
It is known that the compound presents a cytotoxic activity towards normal human pbmc, only at very high concentrations .
Result of Action
The compound causes a reduction in the viability of A549 cells when treated with specific concentrations of the compound for certain durations . It also induces apoptosis in these cells, as evidenced by caspase 3 activation . At low concentrations, the compound induces peripheral blood lymphocytes proliferation and also stimulates them to IL-2 production .
Biochemical Analysis
Biochemical Properties
Cis-Ruthenium Chloride plays a significant role in biochemical reactions. It interacts with various enzymes, proteins, and other biomolecules. For instance, it has been found to interact with triazolopyrimidine-derived ligands in the formation of novel ruthenium (III) complexes .
Cellular Effects
The effects of this compound on various types of cells and cellular processes are quite profound. It has been found to have cytotoxic activity towards normal human peripheral blood mononuclear cells (PBMC) at very high concentrations . At low concentrations, it stimulates proliferation and interleukin-2 (IL-2) production, while at high concentrations, it induces cytotoxicity, inhibits the ability to proliferate, and inhibits IL-2 production .
Molecular Mechanism
The molecular mechanism of this compound involves its interaction with biomolecules at the molecular level. It has been suggested that it binds to the D-loop region of the mitochondrial DNA of cancer stem cells, inhibiting OXPHOS complex-associated transcription, leading to reduced mitochondrial oxygen consumption, membrane potential, and ATP production .
Temporal Effects in Laboratory Settings
In laboratory settings, the effects of this compound change over time. Detailed kinetic studies of the hydrolysis of the complexes in the pH range 5–8 have been carried out to determine the stability of the complexes in solution .
Preparation Methods
Cis-ruthenium chloride is typically synthesized by heating ruthenium trichloride in dimethyl sulfoxide (DMSO) under a hydrogen atmosphere. Modern methods have been developed to avoid the use of hydrogen gas, such as using ascorbic acid or refluxing DMSO to reduce the ruthenium . Another method involves microwave synthesis, which significantly reduces the reaction time .
Chemical Reactions Analysis
Cis-ruthenium chloride undergoes various types of chemical reactions, including:
Oxidation and Reduction: It can participate in redox reactions, often involving changes in the oxidation state of ruthenium.
Substitution: The DMSO ligands in this compound can be substituted with other ligands, such as phosphines or amines, under appropriate conditions.
Coordination Chemistry: It forms complexes with a variety of ligands, which can alter its chemical properties and reactivity.
Common reagents used in these reactions include phosphines, amines, and other coordinating ligands. The major products formed depend on the specific ligands and reaction conditions used.
Scientific Research Applications
Cis-ruthenium chloride has a wide range of applications in scientific research:
Chemistry: It is used as a catalyst in various organic reactions, including hydrogenation and transfer hydrogenation.
Biology and Medicine: It has shown potential as an anticancer agent, with several ruthenium-based compounds undergoing clinical trials
Industry: It is used in the synthesis of fine chemicals and pharmaceuticals due to its catalytic properties.
Comparison with Similar Compounds
Cis-ruthenium chloride can be compared with other ruthenium-based compounds, such as:
Ruthenium(III) chloride: Another commonly used ruthenium compound with different oxidation states and reactivity.
Ruthenium Schiff base complexes: These complexes have applications in catalysis and medicinal chemistry, similar to this compound.
Ruthenium(II)–arene complexes: Known for their anticancer properties and different mechanisms of action compared to this compound.
This compound is unique due to its specific ligand environment and its ability to form stable complexes with a variety of ligands, making it versatile in both chemical and biological applications.
Properties
CAS No. |
59091-96-2 |
---|---|
Molecular Formula |
C8H24Cl2O4RuS4 |
Molecular Weight |
484.5 g/mol |
IUPAC Name |
dichlororuthenium;methylsulfinylmethane |
InChI |
InChI=1S/4C2H6OS.2ClH.Ru/c4*1-4(2)3;;;/h4*1-2H3;2*1H;/q;;;;;;+2/p-2 |
InChI Key |
UMJDEUKQHKMAOI-UHFFFAOYSA-L |
SMILES |
CS(=O)C.CS(=O)C.CS(=O)C.CS(=O)C.[Cl-].[Cl-].[Ru+2] |
Canonical SMILES |
CS(=O)C.CS(=O)C.CS(=O)C.CS(=O)C.Cl[Ru]Cl |
Origin of Product |
United States |
Retrosynthesis Analysis
AI-Powered Synthesis Planning: Our tool employs the Template_relevance Pistachio, Template_relevance Bkms_metabolic, Template_relevance Pistachio_ringbreaker, Template_relevance Reaxys, Template_relevance Reaxys_biocatalysis model, leveraging a vast database of chemical reactions to predict feasible synthetic routes.
One-Step Synthesis Focus: Specifically designed for one-step synthesis, it provides concise and direct routes for your target compounds, streamlining the synthesis process.
Accurate Predictions: Utilizing the extensive PISTACHIO, BKMS_METABOLIC, PISTACHIO_RINGBREAKER, REAXYS, REAXYS_BIOCATALYSIS database, our tool offers high-accuracy predictions, reflecting the latest in chemical research and data.
Strategy Settings
Precursor scoring | Relevance Heuristic |
---|---|
Min. plausibility | 0.01 |
Model | Template_relevance |
Template Set | Pistachio/Bkms_metabolic/Pistachio_ringbreaker/Reaxys/Reaxys_biocatalysis |
Top-N result to add to graph | 6 |
Feasible Synthetic Routes
Disclaimer and Information on In-Vitro Research Products
Please be aware that all articles and product information presented on BenchChem are intended solely for informational purposes. The products available for purchase on BenchChem are specifically designed for in-vitro studies, which are conducted outside of living organisms. In-vitro studies, derived from the Latin term "in glass," involve experiments performed in controlled laboratory settings using cells or tissues. It is important to note that these products are not categorized as medicines or drugs, and they have not received approval from the FDA for the prevention, treatment, or cure of any medical condition, ailment, or disease. We must emphasize that any form of bodily introduction of these products into humans or animals is strictly prohibited by law. It is essential to adhere to these guidelines to ensure compliance with legal and ethical standards in research and experimentation.