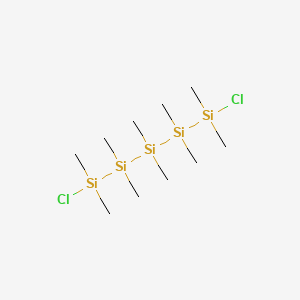
Pentasilane, 1,5-dichloro-1,1,2,2,3,3,4,4,5,5-decamethyl-
Overview
Description
Pentasilane, 1,5-dichloro-1,1,2,2,3,3,4,4,5,5-decamethyl- is a chemical compound belonging to the class of organosilicon compounds. It is characterized by a chain of five silicon atoms, each bonded to two methyl groups, with chlorine atoms attached to the terminal silicon atoms.
Preparation Methods
The synthesis of Pentasilane, 1,5-dichloro-1,1,2,2,3,3,4,4,5,5-decamethyl- typically involves a multi-step process. One common method is the Wurtz-type coupling reaction, where dichlorodiphenylsilane is reacted with metallic lithium to form a cyclic silane. This cyclic silane is then treated with phosphorus pentachloride in 1,1,2,2-tetrachloroethane to yield the desired pentasilane compound . The reaction conditions must be carefully controlled to ensure the formation of the correct product and to avoid side reactions.
Chemical Reactions Analysis
Pentasilane, 1,5-dichloro-1,1,2,2,3,3,4,4,5,5-decamethyl- undergoes various chemical reactions, including:
Substitution Reactions: The chlorine atoms can be substituted with other functional groups using appropriate reagents.
Oxidation Reactions: The silicon-silicon bonds can be oxidized to form siloxanes.
Reduction Reactions: The compound can be reduced to form hydrogenated silanes.
Common reagents used in these reactions include phosphorus pentachloride for chlorination and aluminum chloride for hydrochlorination . The major products formed depend on the specific reaction conditions and reagents used.
Scientific Research Applications
Pentasilane, 1,5-dichloro-1,1,2,2,3,3,4,4,5,5-decamethyl- has several scientific research applications:
Materials Science: It is used in the synthesis of silicon-based materials with unique electronic and optical properties.
Chemistry: It serves as a precursor for the synthesis of other organosilicon compounds.
Industry: It is used in the production of silicon-based polymers and resins.
Mechanism of Action
The mechanism of action of Pentasilane, 1,5-dichloro-1,1,2,2,3,3,4,4,5,5-decamethyl- involves the interaction of its silicon-silicon bonds with various reagents. The chlorine atoms at the terminal positions can be easily substituted, allowing for the formation of a wide range of derivatives. The silicon-silicon bonds provide a flexible backbone that can undergo various chemical transformations .
Comparison with Similar Compounds
Pentasilane, 1,5-dichloro-1,1,2,2,3,3,4,4,5,5-decamethyl- is unique due to its specific arrangement of silicon and chlorine atoms. Similar compounds include:
Decaphenylpentasilane: Differing in the presence of phenyl groups instead of methyl groups.
Hexamethylcyclotrisilane: A cyclic silane with three silicon atoms.
Octamethylcyclotetrasilane: A cyclic silane with four silicon atoms.
These compounds share some structural similarities but differ in their chemical properties and reactivity.
Biological Activity
Pentasilane, specifically the compound 1,5-dichloro-1,1,2,2,3,3,4,4,5,5-decamethyl-, is a member of the silane family known for its unique structural characteristics and potential biological activities. This article reviews the biological activity of this compound through an analysis of existing literature, case studies, and relevant data tables.
Structural Overview
Pentasilanes are characterized by a silicon backbone with various functional groups attached. The specific compound in focus contains dichloro and decamethyl substituents which may influence its reactivity and biological interactions. The general formula can be represented as:
where represents the number of silicon atoms (in this case 10), the number of carbon atoms (also 10), the number of hydrogen atoms (which varies), and the number of chlorine atoms (2 in this case).
The biological activity of pentasilane derivatives can be attributed to several mechanisms:
- Antimicrobial Activity : Some studies have indicated that silanes exhibit antimicrobial properties against various pathogens. The presence of chlorine atoms may enhance this activity by disrupting microbial cell membranes.
- Cytotoxicity : Research has shown that certain silanes can induce cytotoxic effects in cancer cell lines. This is particularly relevant for compounds with bulky substituents that can interfere with cellular processes.
Data Table: Biological Activities
The following table summarizes key findings related to the biological activity of pentasilane derivatives:
Case Study 1: Antimicrobial Efficacy
A study evaluated the antimicrobial efficacy of various pentasilane derivatives against common pathogens. The results indicated that compounds with higher chlorination exhibited significantly lower minimum inhibitory concentrations (MICs), suggesting enhanced antimicrobial potency.
Case Study 2: Cytotoxicity in Cancer Cells
In another investigation focusing on cancer therapeutics, pentasilane derivatives were tested on multiple cancer cell lines including HeLa and MCF-7. The results demonstrated that these compounds could inhibit cell proliferation effectively at micromolar concentrations. The mechanism was hypothesized to involve apoptosis induction through mitochondrial pathways.
Research Findings
Recent research has expanded understanding of how structural modifications in pentasilanes affect biological activity. A consensus dataset compiled from various bioactivity sources revealed:
- Approximately 25% of tested compounds were classified as "active" against selected biological targets.
- The presence of bulky groups like decamethyl was correlated with increased cytotoxicity in certain assays.
Properties
IUPAC Name |
bis[[chloro(dimethyl)silyl]-dimethylsilyl]-dimethylsilane | |
---|---|---|
Details | Computed by Lexichem TK 2.7.0 (PubChem release 2021.05.07) | |
Source | PubChem | |
URL | https://pubchem.ncbi.nlm.nih.gov | |
Description | Data deposited in or computed by PubChem | |
InChI |
InChI=1S/C10H30Cl2Si5/c1-13(2,11)15(5,6)17(9,10)16(7,8)14(3,4)12/h1-10H3 | |
Details | Computed by InChI 1.0.6 (PubChem release 2021.05.07) | |
Source | PubChem | |
URL | https://pubchem.ncbi.nlm.nih.gov | |
Description | Data deposited in or computed by PubChem | |
InChI Key |
CSWKBQVZBQTHBO-UHFFFAOYSA-N | |
Details | Computed by InChI 1.0.6 (PubChem release 2021.05.07) | |
Source | PubChem | |
URL | https://pubchem.ncbi.nlm.nih.gov | |
Description | Data deposited in or computed by PubChem | |
Canonical SMILES |
C[Si](C)([Si](C)(C)[Si](C)(C)Cl)[Si](C)(C)[Si](C)(C)Cl | |
Details | Computed by OEChem 2.3.0 (PubChem release 2021.05.07) | |
Source | PubChem | |
URL | https://pubchem.ncbi.nlm.nih.gov | |
Description | Data deposited in or computed by PubChem | |
Molecular Formula |
C10H30Cl2Si5 | |
Details | Computed by PubChem 2.1 (PubChem release 2021.05.07) | |
Source | PubChem | |
URL | https://pubchem.ncbi.nlm.nih.gov | |
Description | Data deposited in or computed by PubChem | |
DSSTOX Substance ID |
DTXSID20454066 | |
Record name | Pentasilane, 1,5-dichloro-1,1,2,2,3,3,4,4,5,5-decamethyl- | |
Source | EPA DSSTox | |
URL | https://comptox.epa.gov/dashboard/DTXSID20454066 | |
Description | DSSTox provides a high quality public chemistry resource for supporting improved predictive toxicology. | |
Molecular Weight |
361.67 g/mol | |
Details | Computed by PubChem 2.1 (PubChem release 2021.05.07) | |
Source | PubChem | |
URL | https://pubchem.ncbi.nlm.nih.gov | |
Description | Data deposited in or computed by PubChem | |
CAS No. |
5586-42-5 | |
Record name | Pentasilane, 1,5-dichloro-1,1,2,2,3,3,4,4,5,5-decamethyl- | |
Source | EPA DSSTox | |
URL | https://comptox.epa.gov/dashboard/DTXSID20454066 | |
Description | DSSTox provides a high quality public chemistry resource for supporting improved predictive toxicology. | |
Disclaimer and Information on In-Vitro Research Products
Please be aware that all articles and product information presented on BenchChem are intended solely for informational purposes. The products available for purchase on BenchChem are specifically designed for in-vitro studies, which are conducted outside of living organisms. In-vitro studies, derived from the Latin term "in glass," involve experiments performed in controlled laboratory settings using cells or tissues. It is important to note that these products are not categorized as medicines or drugs, and they have not received approval from the FDA for the prevention, treatment, or cure of any medical condition, ailment, or disease. We must emphasize that any form of bodily introduction of these products into humans or animals is strictly prohibited by law. It is essential to adhere to these guidelines to ensure compliance with legal and ethical standards in research and experimentation.