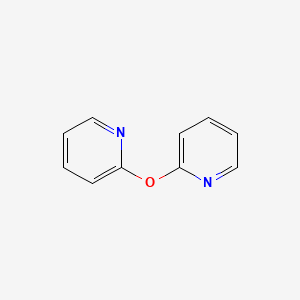
Dipyridyl ether
Overview
Description
Preparation Methods
Synthetic Routes and Reaction Conditions
Dipyridyl ether can be synthesized through several methods. One common approach involves the Ullmann-type reaction, where aryl halides react with copper catalysts to form the ether linkage. Another method is the Buchwald–Hartwig cross-coupling reaction, which uses palladium catalytic systems to couple aryl halides with phenols. Additionally, the Chan–Lam cross-coupling reaction, catalyzed by copper, can be used to couple phenols with arylboronic acids .
Industrial Production Methods
In industrial settings, the production of this compound often involves large-scale catalytic processes. These methods typically employ high-boiling solvents such as glycol dimethyl ether or dibenzyl ether to increase yield and selectivity .
Chemical Reactions Analysis
Types of Reactions
Dipyridyl ether undergoes various chemical reactions, including:
Oxidation: The compound can be oxidized using strong oxidizing agents, leading to the formation of pyridine N-oxide derivatives.
Reduction: Reduction reactions can convert this compound into its corresponding amine derivatives.
Common Reagents and Conditions
Oxidation: Common oxidizing agents include potassium permanganate and chromium trioxide.
Reduction: Reducing agents such as lithium aluminum hydride or sodium borohydride are typically used.
Substitution: Strong acids like hydrobromic acid or hydroiodic acid are employed for cleavage reactions.
Major Products Formed
Oxidation: Pyridine N-oxide derivatives.
Reduction: Amine derivatives.
Substitution: Alkyl halides and phenols.
Scientific Research Applications
Mechanism of Action
The mechanism by which dipyridyl ether exerts its effects involves its ability to chelate metal ions. This chelation process forms stable complexes with transition metals, which can then participate in various catalytic and electron transfer reactions. The molecular targets and pathways involved include the inhibition of phosphodiesterase enzymes, leading to increased levels of cyclic adenosine monophosphate (cAMP) and cyclic guanosine monophosphate (cGMP), which play crucial roles in cellular signaling .
Comparison with Similar Compounds
Similar Compounds
2,2’-Bipyridine: A chelating ligand similar to dipyridyl ether, used in coordination chemistry and catalysis.
4,4’-Bipyridine: Another isomer of bipyridine, primarily used in the manufacture of pesticides and as a precursor to redox-active compounds.
1,10-Phenanthroline: A related compound used as a ligand in coordination chemistry and known for its strong binding affinity to metal ions.
Uniqueness
This compound is unique due to its ether linkage, which imparts distinct chemical properties compared to other bipyridine derivatives. This linkage allows for different reactivity and stability, making it a valuable compound in various chemical and industrial applications.
Properties
IUPAC Name |
2-pyridin-2-yloxypyridine | |
---|---|---|
Details | Computed by Lexichem TK 2.7.0 (PubChem release 2021.05.07) | |
Source | PubChem | |
URL | https://pubchem.ncbi.nlm.nih.gov | |
Description | Data deposited in or computed by PubChem | |
InChI |
InChI=1S/C10H8N2O/c1-3-7-11-9(5-1)13-10-6-2-4-8-12-10/h1-8H | |
Details | Computed by InChI 1.0.6 (PubChem release 2021.05.07) | |
Source | PubChem | |
URL | https://pubchem.ncbi.nlm.nih.gov | |
Description | Data deposited in or computed by PubChem | |
InChI Key |
JMTMWFZXOIWTLX-UHFFFAOYSA-N | |
Details | Computed by InChI 1.0.6 (PubChem release 2021.05.07) | |
Source | PubChem | |
URL | https://pubchem.ncbi.nlm.nih.gov | |
Description | Data deposited in or computed by PubChem | |
Canonical SMILES |
C1=CC=NC(=C1)OC2=CC=CC=N2 | |
Details | Computed by OEChem 2.3.0 (PubChem release 2021.05.07) | |
Source | PubChem | |
URL | https://pubchem.ncbi.nlm.nih.gov | |
Description | Data deposited in or computed by PubChem | |
Molecular Formula |
C10H8N2O | |
Details | Computed by PubChem 2.1 (PubChem release 2021.05.07) | |
Source | PubChem | |
URL | https://pubchem.ncbi.nlm.nih.gov | |
Description | Data deposited in or computed by PubChem | |
DSSTOX Substance ID |
DTXSID20344731 | |
Record name | dipyridyl ether | |
Source | EPA DSSTox | |
URL | https://comptox.epa.gov/dashboard/DTXSID20344731 | |
Description | DSSTox provides a high quality public chemistry resource for supporting improved predictive toxicology. | |
Molecular Weight |
172.18 g/mol | |
Details | Computed by PubChem 2.1 (PubChem release 2021.05.07) | |
Source | PubChem | |
URL | https://pubchem.ncbi.nlm.nih.gov | |
Description | Data deposited in or computed by PubChem | |
CAS No. |
53258-94-9 | |
Record name | dipyridyl ether | |
Source | EPA DSSTox | |
URL | https://comptox.epa.gov/dashboard/DTXSID20344731 | |
Description | DSSTox provides a high quality public chemistry resource for supporting improved predictive toxicology. | |
Retrosynthesis Analysis
AI-Powered Synthesis Planning: Our tool employs the Template_relevance Pistachio, Template_relevance Bkms_metabolic, Template_relevance Pistachio_ringbreaker, Template_relevance Reaxys, Template_relevance Reaxys_biocatalysis model, leveraging a vast database of chemical reactions to predict feasible synthetic routes.
One-Step Synthesis Focus: Specifically designed for one-step synthesis, it provides concise and direct routes for your target compounds, streamlining the synthesis process.
Accurate Predictions: Utilizing the extensive PISTACHIO, BKMS_METABOLIC, PISTACHIO_RINGBREAKER, REAXYS, REAXYS_BIOCATALYSIS database, our tool offers high-accuracy predictions, reflecting the latest in chemical research and data.
Strategy Settings
Precursor scoring | Relevance Heuristic |
---|---|
Min. plausibility | 0.01 |
Model | Template_relevance |
Template Set | Pistachio/Bkms_metabolic/Pistachio_ringbreaker/Reaxys/Reaxys_biocatalysis |
Top-N result to add to graph | 6 |
Feasible Synthetic Routes
Q1: What is the structural significance of the C-O-C bond angle in dipyridyl ethers?
A1: The C-O-C bond angle in dipyridyl ethers provides insights into the molecule's conformation and potential reactivity. In [4,4′-Oxybis(2,6-dimethylpyridinium) bis(trifluoromethanesulfonate)], the C-O-C angle is reported as 119.3(2)°, which is consistent with other dipyridyl ether structures []. This angle, along with the C-O bond distances, suggests a typical ether linkage and provides information for understanding the molecule's overall shape.
Q2: How are dipyridyl ethers used in coordination polymer synthesis?
A2: Dipyridyl ethers, like 3,3’-oxybis(pyridine) (obp) and 1,4-bis(3-pyridoxy)benzene (bpob), act as bridging ligands in the formation of coordination polymers [, ]. These ligands coordinate to metal ions, such as Cobalt(II), through their nitrogen atoms, creating extended 2-dimensional sheet structures. The specific this compound used influences the size and geometry of the resulting metal-organic frameworks.
Q3: What interesting reactivity has been observed with dipyridyl ethers and pyridine-N-oxide?
A3: Research indicates that heating specific isomers of pyridyl p-toluenesulfonate with pyridine-N-oxide leads to the formation of various products, including dipyridyl ethers []. For instance, reacting 2-pyridyl p-toluenesulfonate with pyridine-N-oxide yields N-(2′-pyridyl)-pyridone-2 as the main product, along with N-(4′-pyridyl)-pyridone-2 and 2,3′-dipyridyl ether. This highlights the potential of dipyridyl ethers as building blocks in organic synthesis.
Q4: Are there computational studies on dipyridyl ethers?
A4: Yes, computational methods have been employed to investigate the electronic structure and spectroscopic properties of dipyridyl ethers. For example, the MIM (Molecules in Molecules) method has been used to study the electronic states and π-π* transitions in diphenyl ether, phenoxypyridines, and dipyridyl ethers []. These studies provide valuable insights into the electronic properties and potential applications of these compounds.
Q5: What analytical techniques are used to characterize dipyridyl ethers?
A5: Various analytical techniques are employed for the characterization of dipyridyl ethers. X-ray crystallography is crucial for determining the solid-state structure, including bond lengths, bond angles, and molecular conformation []. Additionally, spectroscopic methods like UV-Vis spectroscopy are used to study electronic transitions and characterize reaction products [].
Disclaimer and Information on In-Vitro Research Products
Please be aware that all articles and product information presented on BenchChem are intended solely for informational purposes. The products available for purchase on BenchChem are specifically designed for in-vitro studies, which are conducted outside of living organisms. In-vitro studies, derived from the Latin term "in glass," involve experiments performed in controlled laboratory settings using cells or tissues. It is important to note that these products are not categorized as medicines or drugs, and they have not received approval from the FDA for the prevention, treatment, or cure of any medical condition, ailment, or disease. We must emphasize that any form of bodily introduction of these products into humans or animals is strictly prohibited by law. It is essential to adhere to these guidelines to ensure compliance with legal and ethical standards in research and experimentation.