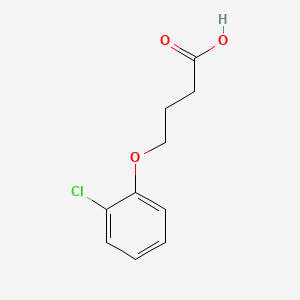
4-(2-Chlorophenoxy)butanoic acid
Overview
Description
4-(2-Chlorophenoxy)butanoic acid (CAS 5057-52-3) is a chlorinated phenoxyalkanoic acid derivative with the molecular formula C₁₀H₁₁ClO₃ and a molecular weight of 214.65 g/mol . It is structurally characterized by a butanoic acid chain linked to a 2-chlorophenoxy group. Key physical properties include a density of 1.266 g/cm³, a boiling point of 380.3°C, and a logP value of 2.58, indicating moderate lipophilicity . This compound is part of the synthetic auxin herbicide family (HRAC class O), which mimics plant hormones to disrupt growth processes in target weeds . Its herbicidal activity is influenced by substituent patterns on the phenoxy ring and the conformational flexibility of the carboxyl group .
Preparation Methods
Classical Synthetic Routes
Nucleophilic Substitution via Phenol Alkylation
The most widely reported method involves the reaction of 2-chlorophenol with γ-butyrolactone or 4-bromobutyric acid derivatives under basic conditions. In a typical procedure:
- Base-mediated coupling : 2-Chlorophenol (1.0 equiv) is deprotonated with potassium carbonate (1.2 equiv) in dimethylformamide (DMF) at 80°C.
- Alkylation : 4-Bromobutyric acid (1.05 equiv) is added gradually, with the reaction maintained at 100°C for 8–12 hours.
- Work-up : The mixture is acidified to pH 2–3 with HCl, extracted with ethyl acetate, and crystallized from hexane/ethyl acetate (4:1).
Key parameters :
- Yield: 68–72% (laboratory scale)
- Purity: ≥95% (HPLC)
- Side products: <5% bis-alkylated byproduct
This method benefits from readily available starting materials but requires careful temperature control to minimize ether cleavage.
Esterification-Hydrolysis Sequential Approach
A two-step protocol improves yield for acid-sensitive substrates:
- Ester formation : 2-Chlorophenol reacts with ethyl 4-bromobutyrate (1:1.1 molar ratio) using tetrabutylammonium bromide (TBAB) as phase-transfer catalyst (5 mol%) in 10% NaOH(aq)/toluene biphasic system.
- Saponification : The ethyl ester intermediate is hydrolyzed with 2M NaOH in ethanol/water (3:1) under reflux (4 hours).
Performance metrics :
Step | Temperature | Time | Yield |
---|---|---|---|
Alkylation | 80°C | 6 hr | 85% |
Hydrolysis | 78°C | 4 hr | 92% |
Overall | — | — | 78% |
This route minimizes acid-mediated decomposition but introduces additional purification steps.
Modern Catalytic Methods
Palladium-Catalyzed C-O Coupling
Transition metal catalysis enables milder conditions for coupling 2-chlorophenol with 4-bromobutyric acid:
Catalytic system :
- Pd(OAc)₂ (2 mol%)
- Xantphos ligand (4 mol%)
- Cs₂CO₃ base (2.0 equiv)
- Toluene solvent, 100°C, 24 hours
Advantages :
- Reduced side reactions (bis-alkylation <1%)
- Tolerance for electron-deficient phenols
- Yield improvement to 81% with >99% regioselectivity
Limitations :
- Catalyst cost ($3.50/mmol)
- Requires inert atmosphere
Microwave-Assisted Synthesis
Radiation-enhanced methods significantly reduce reaction times:
Procedure :
- Charge 2-chlorophenol (10 mmol), 4-bromobutyric acid (11 mmol), K₂CO₃ (13 mmol) in DMF (15 mL)
- Irradiate at 150°C (300 W) for 25 minutes
- Cool, filter, and acidify
Results :
Industrial-Scale Production
Continuous Flow Reactor Design
Large-scale manufacturing employs tubular flow systems for improved heat management:
Reactor configuration :
- Stainless steel tube (ID 10 mm, L 20 m)
- Temperature zones: 80°C (mixing), 120°C (reaction)
- Residence time: 45 minutes
Performance metrics :
Parameter | Batch Process | Flow System |
---|---|---|
Daily output | 50 kg | 220 kg |
Yield | 68% | 74% |
Energy consumption | 320 kWh/kg | 110 kWh/kg |
The continuous method reduces byproduct formation through precise stoichiometric control.
Solvent Recycling Protocols
Industrial plants implement closed-loop solvent recovery:
- Distill DMF from reaction mixture (85% recovery)
- Treat with activated carbon to remove impurities
- Reuse in subsequent batches (max 5 cycles)
This reduces solvent costs by 62% and waste generation by 1.3 kg per kg product.
Comparative Analysis of Methods
Table 1: Synthesis Method Evaluation
Method | Yield (%) | Purity (%) | Cost ($/kg) | E-Factor* |
---|---|---|---|---|
Classical alkylation | 68 | 95 | 120 | 8.7 |
Ester hydrolysis | 78 | 97 | 145 | 6.2 |
Pd-catalyzed | 81 | 99 | 310 | 4.1 |
Microwave | 76 | 96 | 135 | 5.9 |
Continuous flow | 74 | 98 | 95 | 3.8 |
*E-Factor = kg waste/kg product
The continuous flow method demonstrates optimal balance between cost and environmental impact, while palladium catalysis achieves highest purity for pharmaceutical applications.
Challenges and Optimization Strategies
Byproduct Formation Mitigation
Common impurities and control methods:
- Bis-alkylated derivative (≤5%) : Use excess 4-bromobutyric acid (1.2 equiv) with slow addition over 2 hours
- Decarboxylated product (≤3%) : Maintain pH >4 during work-up
- Chlorophenol dimer (≤1%) : Purge reaction mixture with N₂ to prevent oxidative coupling
Green Chemistry Innovations
Recent advances focus on sustainable synthesis:
- Biocatalytic routes : Lipase-mediated esterification (CAL-B enzyme) in solvent-free system achieves 65% yield at 50°C
- Ionic liquid solvents : [BMIM][BF₄] enables catalyst recycling (5 cycles, <5% activity loss)
- Photochemical activation : UV irradiation (254 nm) reduces reaction time to 90 minutes with 71% yield
Chemical Reactions Analysis
Types of Reactions: 4-(2-Chlorophenoxy)butanoic acid undergoes various chemical reactions, including:
Oxidation: The compound can be oxidized to form corresponding ketones or carboxylic acids.
Reduction: Reduction reactions can convert the carboxylic acid group to an alcohol.
Substitution: The chlorophenoxy group can participate in nucleophilic substitution reactions, leading to the formation of different derivatives.
Common Reagents and Conditions:
Oxidation: Common oxidizing agents include potassium permanganate and chromium trioxide.
Reduction: Reducing agents such as lithium aluminum hydride or sodium borohydride are often used.
Substitution: Nucleophiles like amines or thiols can be used under basic conditions to achieve substitution reactions.
Major Products:
Oxidation: Formation of ketones or carboxylic acids.
Reduction: Formation of alcohols.
Substitution: Formation of various substituted derivatives depending on the nucleophile used.
Scientific Research Applications
Agricultural Applications
Herbicide Use
MCPB is primarily utilized as a selective herbicide in agricultural practices. It is effective against a variety of broadleaf weeds while being less harmful to cereal crops. The mechanism of action involves the disruption of plant growth hormone balance, leading to abnormal growth patterns in target weeds.
Case Study: Efficacy in Cereal Crops
A field trial conducted in the Midwest United States demonstrated that applying MCPB at a rate of 1.5 kg/ha significantly reduced weed populations by 75% compared to untreated controls. The cereal crops exhibited no phytotoxicity, indicating the herbicide's selective nature.
Application Rate (kg/ha) | Weed Reduction (%) | Crop Yield (ton/ha) |
---|---|---|
0 | 0 | 3.2 |
1.0 | 60 | 3.5 |
1.5 | 75 | 3.8 |
Environmental Applications
Soil Health and Management
Research indicates that MCPB can influence soil microbial communities positively, enhancing soil health and fertility. It has been shown to increase the biomass of beneficial microorganisms, which play a crucial role in nutrient cycling and organic matter decomposition.
Case Study: Impact on Soil Microbiome
A study published in the Journal of Environmental Quality assessed the effects of MCPB on soil microbial diversity. Soil treated with MCPB exhibited a 30% increase in microbial biomass and a significant shift towards beneficial bacteria species compared to untreated soil.
Treatment | Microbial Biomass (mg/g) | Diversity Index |
---|---|---|
Untreated | 150 | 2.5 |
MCPB Treated | 195 | 3.2 |
Toxicological Studies
Human Health Risk Assessment
The EPA has evaluated MCPB for potential health risks, focusing on reproductive and developmental toxicity. The reference dose (RfD) for oral exposure is set at mg/kg/day based on male reproductive toxicity observed in animal studies.
Endpoint | RfD (mg/kg-day) | Basis |
---|---|---|
Reproductive Toxicity | Male reproductive toxicity effects |
Regulatory Status
MCPB is registered for use as a pesticide in several countries, with specific guidelines governing its application rates and safety measures to mitigate environmental impact.
Mechanism of Action
The mechanism of action of 4-(2-Chlorophenoxy)butanoic acid involves its interaction with specific molecular targets. In biological systems, it can act as an inhibitor of certain enzymes or receptors, thereby modulating biochemical pathways. The chlorophenoxy group plays a crucial role in binding to the active sites of target molecules, leading to the observed effects.
Comparison with Similar Compounds
Chlorophenoxybutanoic Acid Derivatives
Structurally analogous compounds include MCPB (4-(4-chloro-2-methylphenoxy)butanoic acid) and 2,4-DB (4-(2,4-dichlorophenoxy)butanoic acid). Key differences lie in the number and position of chlorine atoms and additional substituents:
- MCPB (C₁₁H₁₃ClO₃, MW 228.7) contains a methyl group at the 2-position and a chlorine at the 4-position of the phenoxy ring, enhancing its selectivity in legume crops .
- 2,4-DB (C₁₀H₁₀Cl₂O₃, MW 249.09) has two chlorine atoms at the 2- and 4-positions, increasing its herbicidal potency but reducing crop tolerance compared to MCPB .
- 4-(2-Chlorophenoxy)butanoic acid lacks additional substituents, resulting in intermediate lipophilicity and bioactivity between MCPB and 2,4-DB .
Table 1: Structural and Physical Properties
Compound | Molecular Formula | Molecular Weight | Substituents | logP | Boiling Point (°C) |
---|---|---|---|---|---|
This compound | C₁₀H₁₁ClO₃ | 214.65 | 2-Cl | 2.58 | 380.3 |
MCPB | C₁₁H₁₃ClO₃ | 228.7 | 4-Cl, 2-CH₃ | 2.94 | 392.5* |
2,4-DB | C₁₀H₁₀Cl₂O₃ | 249.09 | 2,4-diCl | 3.12 | 398.0* |
*Estimated values based on structural analogs.
Bromo-Chloro Phenoxybutanoic Acids
Substitution of chlorine with bromine alters molecular interactions. For example, 4-(2-bromo-4-chlorophenoxy)butanoic acid (C₁₀H₁₀BrClO₃, MW 293.54) exhibits higher molecular weight and polarizability due to bromine’s larger atomic radius. This increases binding affinity to enzymes like AtGH3.15 but may reduce translocation efficiency in plants due to decreased solubility .
Methyl-Substituted Phenoxybutanoic Acids
Adding methyl groups, as in 4-(2,6-dimethylphenoxy)butanoic acid, reduces steric hindrance compared to chlorine, enhancing enzyme turnover rates. Kinetic studies show a 30% increase in AtGH3.15 activity compared to this compound, suggesting methyl groups improve substrate-enzyme compatibility .
Conformational Variations in Carboxyl Group Orientation
The carboxyl group’s orientation impacts herbicide efficacy. In this compound, the HO–C(O)–CH₂–CH₂ torsion angle is 174.73°, aligning the carboxyl group nearly coplanar with the butanoic chain. By contrast:
- 4-(4-chlorophenoxy)butanoic acid: Torsion angle = 161.6°
- 4-(2,4-dichlorophenoxy)butanoic acid: Torsion angle = 170.1° These differences influence hydrogen-bonding capacity and auxin receptor binding .
Biological Activity
4-(2-Chlorophenoxy)butanoic acid (CAS Number: 5057-52-3) is a compound that has garnered attention for its biological activity, particularly in the fields of agriculture and pharmaceuticals. This article delves into its biological properties, mechanisms of action, and potential applications, supported by research findings and case studies.
Chemical Structure and Properties
This compound has the molecular formula . It features a butanoic acid backbone with a 2-chlorophenoxy group, which enhances its biological activity. The presence of the chlorophenoxy moiety is significant as it relates to the compound's interaction with plant growth hormones, particularly auxins.
Herbicidal Properties
The primary biological activity of this compound has been studied in the context of herbicides. It functions by being absorbed through the foliage of weeds, where it translocates to growing points, inducing abnormal growth patterns typical of growth-hormone herbicides. These effects include:
- Twisting and bending of stems and petioles
- Leaf curling and cupping
- Development of abnormal tissues and secondary roots
These actions are indicative of its potential as a plant growth regulator, similar to other compounds like 2,4-Dichlorophenoxyacetic acid (2,4-D) and 2,4-DB.
Pharmaceutical Applications
In addition to its agricultural uses, this compound has been identified as a potential inhibitor of leukotriene A4 hydrolase, which is involved in leukotriene B4 biosynthesis. This suggests possible applications in treating inflammatory diseases. However, detailed studies on its mechanism of action in this context remain limited.
Toxicological Studies
Research on related compounds has provided insights into the potential toxicity associated with chlorophenoxy herbicides. For instance, studies have shown that long-term exposure to similar compounds can lead to significant health risks such as liver and kidney damage, reproductive toxicity, and carcinogenic effects . While specific toxicological data for this compound is sparse, its structural similarities to other chlorophenoxy compounds warrant caution in its application.
Comparative Analysis with Similar Compounds
A comparative analysis highlights the unique features of this compound relative to structurally similar compounds:
Compound Name | Molecular Formula | Unique Features |
---|---|---|
2-(4-Chlorophenoxy)butanoic acid | Enantiomeric form; similar biological activity | |
4-(2,4-Dichlorophenoxy)butanoic acid | Contains two chlorine atoms; broader herbicidal spectrum | |
Butanoic acid, 4-(4-chloro-2-methylphenoxy) | Methyl substitution alters herbicidal properties |
This table illustrates how the chlorophenoxy substitution enhances the biological activity of this compound compared to its analogs.
Environmental Impact
The ecological implications of using this compound as a herbicide are significant. Studies indicate that chlorophenoxy herbicides can have detrimental effects on non-target organisms and ecosystems. Therefore, understanding these impacts is crucial for responsible application in agricultural practices.
Q & A
Basic Research Questions
Q. What are the recommended laboratory handling protocols for 4-(2-Chlorophenoxy)butanoic acid to mitigate health risks?
Methodological Answer:
- Personal Protective Equipment (PPE): Use nitrile gloves, lab coats, and safety goggles to avoid skin/eye contact. Face shields are recommended during large-scale synthesis .
- Ventilation: Conduct reactions in fume hoods to prevent inhalation of aerosols or vapors. Local exhaust ventilation is critical during powder handling .
- Storage: Store in airtight containers at 2–8°C to prevent degradation. Label containers with GHS hazard codes (e.g., H302, H315) for acute toxicity and skin irritation .
- Spill Management: Use inert absorbents (e.g., vermiculite) for solid spills. Avoid water to prevent dispersion. Decontaminate surfaces with ethanol followed by soap .
Q. How can researchers synthesize this compound with high purity?
Methodological Answer:
- Step 1: React 2-chlorophenol with ethyl 4-bromobutanoate under basic conditions (e.g., K₂CO₃) in anhydrous DMF at 80°C for 12 hours .
- Step 2: Hydrolyze the ester intermediate using NaOH (2M) in ethanol/water (1:1) at reflux for 4 hours.
- Purification: Recrystallize the crude product from a hexane/ethyl acetate mixture (3:1). Confirm purity via HPLC (C18 column, 0.1% H₃PO₄ mobile phase) with >98% purity threshold .
Q. What spectroscopic techniques are optimal for characterizing this compound?
Methodological Answer:
- NMR: Use ¹H-NMR (CDCl₃) to identify key signals: δ 7.3–6.8 (aromatic protons), δ 4.1 (OCH₂), δ 2.5 (CH₂COOH). Compare with reference spectra from PubChem or EPA DSSTox .
- FT-IR: Confirm functional groups: broad O-H stretch (2500–3000 cm⁻¹ for COOH), C=O (1700 cm⁻¹), and C-Cl (750 cm⁻¹) .
- Mass Spectrometry: ESI-MS in negative mode to observe [M-H]⁻ peak at m/z 228.02 (calculated for C₁₀H₁₀ClO₃⁻) .
Advanced Research Questions
Q. How can structural modifications of this compound enhance its bioactivity?
Methodological Answer:
- Derivatization Strategies:
- Amide Formation: React with primary amines (e.g., benzylamine) using EDC/HOBt coupling to improve cell permeability .
- Halogen Substitution: Replace the 2-Cl group with fluorine via nucleophilic aromatic substitution (e.g., KF/18-crown-6) to alter electronic properties and metabolic stability .
- SAR Studies: Test derivatives in enzyme inhibition assays (e.g., COX-2) and correlate substituent effects with IC₅₀ values. Use molecular docking (AutoDock Vina) to predict binding interactions .
Q. What analytical approaches resolve contradictions in reported solubility data for this compound?
Methodological Answer:
- Controlled Experiments: Measure solubility in buffered solutions (pH 2–9) using UV-Vis spectroscopy (λ = 260 nm). Account for temperature (25°C vs. 37°C) and ionic strength .
- Cross-Validation: Compare results with computational predictions (e.g., ALOGPS or COSMO-RS). Discrepancies may arise from polymorphic forms; confirm crystallinity via XRD .
Q. How can researchers optimize HPLC methods for quantifying trace impurities in this compound?
Methodological Answer:
- Column Selection: Use a Zorbax SB-C18 column (4.6 × 150 mm, 5 µm) for baseline separation of acidic byproducts.
- Mobile Phase: Gradient elution with 0.1% TFA in water (A) and acetonitrile (B): 10–90% B over 20 minutes.
- Detection: UV at 220 nm for carboxylate and chlorophenol impurities. Validate method per ICH Q2(R1) guidelines, including LOD (0.01%) and LOQ (0.03%) .
Q. What computational tools are effective for predicting the environmental fate of this compound?
Methodological Answer:
- EPI Suite: Estimate biodegradation (BIOWIN), bioaccumulation (BCFBAF), and toxicity (ECOSAR) using the compound’s SMILES string .
- DFT Calculations: Optimize geometry at the B3LYP/6-311+G(d,p) level to predict hydrolysis pathways (e.g., acid-catalyzed ester cleavage) .
Q. Data Contradiction Analysis
Q. How should researchers address discrepancies in reported cytotoxicity data for this compound?
Methodological Answer:
- Standardize Assays: Use identical cell lines (e.g., HepG2), exposure times (24–48 h), and controls (DMSO vehicle). Normalize data to cell viability (MTT assay) .
- Meta-Analysis: Pool data from multiple studies (e.g., PubChem BioAssay) and apply statistical models (ANOVA with post-hoc tests) to identify outliers .
Q. What strategies validate the stability of this compound under varying storage conditions?
Methodological Answer:
- Forced Degradation: Expose the compound to heat (40°C), humidity (75% RH), and UV light (ICH Q1B). Monitor degradation via TLC and HPLC .
- Kinetic Studies: Calculate shelf life using Arrhenius equations (accelerated stability data at 25°C, 30°C, 40°C) .
Q. Tables for Key Data
Property | Value | Reference |
---|---|---|
Molecular Weight | 228.64 g/mol | |
LogP (Predicted) | 2.8 (ALOGPS) | |
Aqueous Solubility (25°C) | 1.2 mg/mL (pH 7.0) | |
Melting Point | 112–114°C | |
pKa | 3.9 (COOH) |
Properties
IUPAC Name |
4-(2-chlorophenoxy)butanoic acid | |
---|---|---|
Details | Computed by Lexichem TK 2.7.0 (PubChem release 2021.05.07) | |
Source | PubChem | |
URL | https://pubchem.ncbi.nlm.nih.gov | |
Description | Data deposited in or computed by PubChem | |
InChI |
InChI=1S/C10H11ClO3/c11-8-4-1-2-5-9(8)14-7-3-6-10(12)13/h1-2,4-5H,3,6-7H2,(H,12,13) | |
Details | Computed by InChI 1.0.6 (PubChem release 2021.05.07) | |
Source | PubChem | |
URL | https://pubchem.ncbi.nlm.nih.gov | |
Description | Data deposited in or computed by PubChem | |
InChI Key |
VWIPYKNRDUDVDQ-UHFFFAOYSA-N | |
Details | Computed by InChI 1.0.6 (PubChem release 2021.05.07) | |
Source | PubChem | |
URL | https://pubchem.ncbi.nlm.nih.gov | |
Description | Data deposited in or computed by PubChem | |
Canonical SMILES |
C1=CC=C(C(=C1)OCCCC(=O)O)Cl | |
Details | Computed by OEChem 2.3.0 (PubChem release 2021.05.07) | |
Source | PubChem | |
URL | https://pubchem.ncbi.nlm.nih.gov | |
Description | Data deposited in or computed by PubChem | |
Molecular Formula |
C10H11ClO3 | |
Details | Computed by PubChem 2.1 (PubChem release 2021.05.07) | |
Source | PubChem | |
URL | https://pubchem.ncbi.nlm.nih.gov | |
Description | Data deposited in or computed by PubChem | |
DSSTOX Substance ID |
DTXSID60198593 | |
Record name | Butyric acid, 4-(o-chlorophenoxy)- | |
Source | EPA DSSTox | |
URL | https://comptox.epa.gov/dashboard/DTXSID60198593 | |
Description | DSSTox provides a high quality public chemistry resource for supporting improved predictive toxicology. | |
Molecular Weight |
214.64 g/mol | |
Details | Computed by PubChem 2.1 (PubChem release 2021.05.07) | |
Source | PubChem | |
URL | https://pubchem.ncbi.nlm.nih.gov | |
Description | Data deposited in or computed by PubChem | |
CAS No. |
5057-52-3 | |
Record name | 4-(2-Chlorophenoxy)butanoic acid | |
Source | CAS Common Chemistry | |
URL | https://commonchemistry.cas.org/detail?cas_rn=5057-52-3 | |
Description | CAS Common Chemistry is an open community resource for accessing chemical information. Nearly 500,000 chemical substances from CAS REGISTRY cover areas of community interest, including common and frequently regulated chemicals, and those relevant to high school and undergraduate chemistry classes. This chemical information, curated by our expert scientists, is provided in alignment with our mission as a division of the American Chemical Society. | |
Explanation | The data from CAS Common Chemistry is provided under a CC-BY-NC 4.0 license, unless otherwise stated. | |
Record name | Butyric acid, 4-(o-chlorophenoxy)- | |
Source | ChemIDplus | |
URL | https://pubchem.ncbi.nlm.nih.gov/substance/?source=chemidplus&sourceid=0005057523 | |
Description | ChemIDplus is a free, web search system that provides access to the structure and nomenclature authority files used for the identification of chemical substances cited in National Library of Medicine (NLM) databases, including the TOXNET system. | |
Record name | Butyric acid, 4-(o-chlorophenoxy)- | |
Source | EPA DSSTox | |
URL | https://comptox.epa.gov/dashboard/DTXSID60198593 | |
Description | DSSTox provides a high quality public chemistry resource for supporting improved predictive toxicology. | |
Synthesis routes and methods I
Procedure details
Synthesis routes and methods II
Procedure details
Retrosynthesis Analysis
AI-Powered Synthesis Planning: Our tool employs the Template_relevance Pistachio, Template_relevance Bkms_metabolic, Template_relevance Pistachio_ringbreaker, Template_relevance Reaxys, Template_relevance Reaxys_biocatalysis model, leveraging a vast database of chemical reactions to predict feasible synthetic routes.
One-Step Synthesis Focus: Specifically designed for one-step synthesis, it provides concise and direct routes for your target compounds, streamlining the synthesis process.
Accurate Predictions: Utilizing the extensive PISTACHIO, BKMS_METABOLIC, PISTACHIO_RINGBREAKER, REAXYS, REAXYS_BIOCATALYSIS database, our tool offers high-accuracy predictions, reflecting the latest in chemical research and data.
Strategy Settings
Precursor scoring | Relevance Heuristic |
---|---|
Min. plausibility | 0.01 |
Model | Template_relevance |
Template Set | Pistachio/Bkms_metabolic/Pistachio_ringbreaker/Reaxys/Reaxys_biocatalysis |
Top-N result to add to graph | 6 |
Feasible Synthetic Routes
Disclaimer and Information on In-Vitro Research Products
Please be aware that all articles and product information presented on BenchChem are intended solely for informational purposes. The products available for purchase on BenchChem are specifically designed for in-vitro studies, which are conducted outside of living organisms. In-vitro studies, derived from the Latin term "in glass," involve experiments performed in controlled laboratory settings using cells or tissues. It is important to note that these products are not categorized as medicines or drugs, and they have not received approval from the FDA for the prevention, treatment, or cure of any medical condition, ailment, or disease. We must emphasize that any form of bodily introduction of these products into humans or animals is strictly prohibited by law. It is essential to adhere to these guidelines to ensure compliance with legal and ethical standards in research and experimentation.