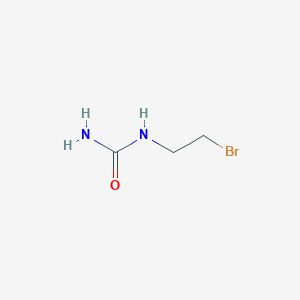
1-(2-Bromoethyl)urea
Overview
Description
1-(2-Bromoethyl)urea is an organic compound with the molecular formula C3H7BrN2O It is a derivative of urea, where one of the hydrogen atoms is replaced by a 2-bromoethyl group
Preparation Methods
Synthetic Routes and Reaction Conditions
1-(2-Bromoethyl)urea can be synthesized through several methods. One common approach involves the reaction of urea with 2-bromoethanol in the presence of a base such as potassium carbonate. The reaction typically proceeds under mild conditions, with the base facilitating the nucleophilic substitution of the hydroxyl group by the urea moiety.
Another method involves the reaction of urea with 2-bromoethylamine hydrobromide. This reaction is usually carried out in an aqueous medium, with the hydrobromide salt providing the necessary bromine source for the substitution reaction.
Industrial Production Methods
Industrial production of this compound typically involves large-scale synthesis using the aforementioned methods. The reactions are optimized for high yield and purity, often employing continuous flow reactors to ensure consistent product quality. The use of automated systems and advanced purification techniques, such as recrystallization and chromatography, further enhances the efficiency of the production process.
Chemical Reactions Analysis
Types of Reactions
1-(2-Bromoethyl)urea undergoes various chemical reactions, including:
Nucleophilic Substitution: The bromine atom in the 2-bromoethyl group can be replaced by other nucleophiles, such as amines, thiols, and alkoxides.
Oxidation: The compound can be oxidized to form corresponding carbonyl compounds, such as aldehydes and carboxylic acids.
Reduction: Reduction of this compound can yield the corresponding amine derivatives.
Common Reagents and Conditions
Nucleophilic Substitution: Common reagents include sodium azide, potassium thiocyanate, and sodium methoxide. The reactions are typically carried out in polar aprotic solvents like dimethyl sulfoxide (DMSO) or acetonitrile.
Oxidation: Oxidizing agents such as potassium permanganate or chromium trioxide are used under acidic or basic conditions.
Reduction: Reducing agents like lithium aluminum hydride (LiAlH4) or sodium borohydride (NaBH4) are employed under anhydrous conditions.
Major Products Formed
Nucleophilic Substitution: Products include azidoethylurea, thiocyanatoethylurea, and methoxyethylurea.
Oxidation: Products include 2-bromoacetaldehyde and 2-bromoacetic acid.
Reduction: Products include 2-aminoethylurea.
Scientific Research Applications
1-(2-Bromoethyl)urea has several scientific research applications:
Chemistry: It is used as an intermediate in the synthesis of various organic compounds, including pharmaceuticals and agrochemicals.
Biology: The compound is studied for its potential biological activity, including antimicrobial and anticancer properties.
Medicine: Research is ongoing to explore its use as a building block for drug development, particularly in the design of novel therapeutic agents.
Industry: It is used in the production of specialty chemicals and as a reagent in various industrial processes.
Mechanism of Action
The mechanism of action of 1-(2-Bromoethyl)urea involves its interaction with biological molecules through nucleophilic substitution reactions. The bromine atom in the 2-bromoethyl group is highly reactive and can form covalent bonds with nucleophilic sites in proteins, DNA, and other biomolecules. This reactivity underlies its potential biological activity, including its antimicrobial and anticancer effects.
Comparison with Similar Compounds
1-(2-Bromoethyl)urea can be compared with other similar compounds, such as:
2-Bromoethylamine: Similar in structure but lacks the urea moiety, making it less versatile in certain synthetic applications.
2-Chloroethylurea: Similar in structure but with a chlorine atom instead of bromine, which affects its reactivity and biological activity.
2-Iodoethylurea:
The uniqueness of this compound lies in its balanced reactivity and stability, making it a valuable compound for various research and industrial applications.
Biological Activity
1-(2-Bromoethyl)urea is a compound of significant interest in the fields of medicinal chemistry and pharmacology due to its potential biological activities, particularly its antimicrobial and anticancer properties. This article provides a comprehensive overview of the biological activity of this compound, supported by various research findings, case studies, and data tables.
This compound is characterized by a bromoethyl group attached to a urea moiety. The bromine atom in the bromoethyl group is highly reactive, allowing it to participate in nucleophilic substitution reactions with biological macromolecules. This reactivity can lead to the formation of covalent bonds with nucleophilic sites in proteins, DNA, and other biomolecules, which underlies its potential biological effects.
Antimicrobial Activity
Research has indicated that this compound exhibits antimicrobial properties. A study evaluated its effectiveness against various bacterial strains, demonstrating significant inhibitory effects. The minimum inhibitory concentrations (MICs) for several Gram-positive and Gram-negative bacteria were determined, showcasing its broad-spectrum activity.
Bacterial Strain | MIC (µg/mL) |
---|---|
Staphylococcus aureus (MRSA) | 16 |
Escherichia coli | 32 |
Enterococcus faecium | 8 |
Pseudomonas aeruginosa | 64 |
These results suggest that this compound could be a candidate for further development as an antimicrobial agent .
Anticancer Properties
The anticancer potential of this compound has also been explored. In vitro studies have shown that it can inhibit the proliferation of various cancer cell lines. For instance, it was tested against human lung cancer (EKVX), leukemia (RPMI-8226), and breast cancer (MDA-MB-435) cell lines.
Cell Line | GI50 (µM) |
---|---|
EKVX | 25.1 |
RPMI-8226 | 21.5 |
MDA-MB-435 | 15.9 |
The compound displayed selective cytotoxicity towards cancer cells while showing lower toxicity towards normal cells, indicating its potential as a therapeutic agent in oncology .
Case Studies
Several case studies have been documented regarding the use of this compound in drug development:
- Study on Antitumor Activity : A series of derivatives based on this compound were synthesized and tested for their antitumor activity. The derivatives showed varying degrees of activity against different cancer cell lines, with some exhibiting significant potency comparable to established chemotherapeutics .
- Mechanistic Insights : Molecular docking studies have provided insights into how this compound interacts with target proteins involved in cancer progression. These studies suggest that the compound may inhibit key enzymatic pathways critical for tumor growth and metastasis .
Properties
IUPAC Name |
2-bromoethylurea | |
---|---|---|
Details | Computed by LexiChem 2.6.6 (PubChem release 2019.06.18) | |
Source | PubChem | |
URL | https://pubchem.ncbi.nlm.nih.gov | |
Description | Data deposited in or computed by PubChem | |
InChI |
InChI=1S/C3H7BrN2O/c4-1-2-6-3(5)7/h1-2H2,(H3,5,6,7) | |
Details | Computed by InChI 1.0.5 (PubChem release 2019.06.18) | |
Source | PubChem | |
URL | https://pubchem.ncbi.nlm.nih.gov | |
Description | Data deposited in or computed by PubChem | |
InChI Key |
ZGGYEZGQRAXKSY-UHFFFAOYSA-N | |
Details | Computed by InChI 1.0.5 (PubChem release 2019.06.18) | |
Source | PubChem | |
URL | https://pubchem.ncbi.nlm.nih.gov | |
Description | Data deposited in or computed by PubChem | |
Canonical SMILES |
C(CBr)NC(=O)N | |
Details | Computed by OEChem 2.1.5 (PubChem release 2019.06.18) | |
Source | PubChem | |
URL | https://pubchem.ncbi.nlm.nih.gov | |
Description | Data deposited in or computed by PubChem | |
Molecular Formula |
C3H7BrN2O | |
Details | Computed by PubChem 2.1 (PubChem release 2019.06.18) | |
Source | PubChem | |
URL | https://pubchem.ncbi.nlm.nih.gov | |
Description | Data deposited in or computed by PubChem | |
DSSTOX Substance ID |
DTXSID50294795 | |
Record name | 1-(2-bromoethyl)urea | |
Source | EPA DSSTox | |
URL | https://comptox.epa.gov/dashboard/DTXSID50294795 | |
Description | DSSTox provides a high quality public chemistry resource for supporting improved predictive toxicology. | |
Molecular Weight |
167.00 g/mol | |
Details | Computed by PubChem 2.1 (PubChem release 2021.05.07) | |
Source | PubChem | |
URL | https://pubchem.ncbi.nlm.nih.gov | |
Description | Data deposited in or computed by PubChem | |
CAS No. |
40380-05-0 | |
Record name | N-(2-Bromoethyl)urea | |
Source | CAS Common Chemistry | |
URL | https://commonchemistry.cas.org/detail?cas_rn=40380-05-0 | |
Description | CAS Common Chemistry is an open community resource for accessing chemical information. Nearly 500,000 chemical substances from CAS REGISTRY cover areas of community interest, including common and frequently regulated chemicals, and those relevant to high school and undergraduate chemistry classes. This chemical information, curated by our expert scientists, is provided in alignment with our mission as a division of the American Chemical Society. | |
Explanation | The data from CAS Common Chemistry is provided under a CC-BY-NC 4.0 license, unless otherwise stated. | |
Record name | NSC 98137 | |
Source | ChemIDplus | |
URL | https://pubchem.ncbi.nlm.nih.gov/substance/?source=chemidplus&sourceid=0040380050 | |
Description | ChemIDplus is a free, web search system that provides access to the structure and nomenclature authority files used for the identification of chemical substances cited in National Library of Medicine (NLM) databases, including the TOXNET system. | |
Record name | NSC98137 | |
Source | DTP/NCI | |
URL | https://dtp.cancer.gov/dtpstandard/servlet/dwindex?searchtype=NSC&outputformat=html&searchlist=98137 | |
Description | The NCI Development Therapeutics Program (DTP) provides services and resources to the academic and private-sector research communities worldwide to facilitate the discovery and development of new cancer therapeutic agents. | |
Explanation | Unless otherwise indicated, all text within NCI products is free of copyright and may be reused without our permission. Credit the National Cancer Institute as the source. | |
Record name | 1-(2-bromoethyl)urea | |
Source | EPA DSSTox | |
URL | https://comptox.epa.gov/dashboard/DTXSID50294795 | |
Description | DSSTox provides a high quality public chemistry resource for supporting improved predictive toxicology. | |
Retrosynthesis Analysis
AI-Powered Synthesis Planning: Our tool employs the Template_relevance Pistachio, Template_relevance Bkms_metabolic, Template_relevance Pistachio_ringbreaker, Template_relevance Reaxys, Template_relevance Reaxys_biocatalysis model, leveraging a vast database of chemical reactions to predict feasible synthetic routes.
One-Step Synthesis Focus: Specifically designed for one-step synthesis, it provides concise and direct routes for your target compounds, streamlining the synthesis process.
Accurate Predictions: Utilizing the extensive PISTACHIO, BKMS_METABOLIC, PISTACHIO_RINGBREAKER, REAXYS, REAXYS_BIOCATALYSIS database, our tool offers high-accuracy predictions, reflecting the latest in chemical research and data.
Strategy Settings
Precursor scoring | Relevance Heuristic |
---|---|
Min. plausibility | 0.01 |
Model | Template_relevance |
Template Set | Pistachio/Bkms_metabolic/Pistachio_ringbreaker/Reaxys/Reaxys_biocatalysis |
Top-N result to add to graph | 6 |
Feasible Synthetic Routes
Disclaimer and Information on In-Vitro Research Products
Please be aware that all articles and product information presented on BenchChem are intended solely for informational purposes. The products available for purchase on BenchChem are specifically designed for in-vitro studies, which are conducted outside of living organisms. In-vitro studies, derived from the Latin term "in glass," involve experiments performed in controlled laboratory settings using cells or tissues. It is important to note that these products are not categorized as medicines or drugs, and they have not received approval from the FDA for the prevention, treatment, or cure of any medical condition, ailment, or disease. We must emphasize that any form of bodily introduction of these products into humans or animals is strictly prohibited by law. It is essential to adhere to these guidelines to ensure compliance with legal and ethical standards in research and experimentation.