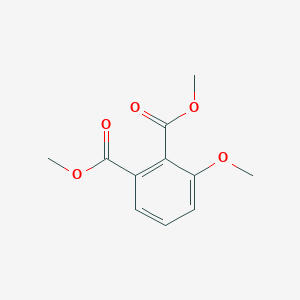
Dimethyl 3-methoxyphthalate
Overview
Description
Dimethyl 3-methoxyphthalate is an organic compound with the molecular formula C11H12O5. It is a dimethyl ester of 3-methoxyphthalic acid and is used in various chemical applications due to its unique properties. The compound is known for its role in organic synthesis and as an intermediate in the production of other chemical substances .
Preparation Methods
Dimethyl 3-methoxyphthalate can be synthesized through several methods. One common synthetic route involves the reaction of 3-methoxyphthalic acid with methanol in the presence of a catalyst such as sulfuric acid. The reaction typically occurs under reflux conditions, resulting in the formation of this compound . Industrial production methods may involve similar esterification processes but on a larger scale, with optimized reaction conditions to maximize yield and purity .
Chemical Reactions Analysis
Dimethyl 3-methoxyphthalate undergoes various chemical reactions, including:
Oxidation: The compound can be oxidized to form 3-methoxyphthalic acid using oxidizing agents such as potassium permanganate.
Reduction: Reduction reactions can convert this compound to its corresponding alcohols using reducing agents like lithium aluminum hydride.
Substitution: The methoxy group in the compound can undergo nucleophilic substitution reactions, leading to the formation of different derivatives.
Common reagents and conditions used in these reactions include strong acids or bases, oxidizing agents, and reducing agents. The major products formed depend on the specific reaction conditions and reagents used .
Scientific Research Applications
Dimethyl 3-methoxyphthalate has several scientific research applications:
Chemistry: It is used as an intermediate in organic synthesis, particularly in the preparation of more complex molecules.
Biology: The compound is studied for its potential biological activities and interactions with various biomolecules.
Medicine: Research is ongoing to explore its potential therapeutic applications, including its role as a precursor in the synthesis of pharmaceutical compounds.
Industry: This compound is used in the production of polymers, resins, and other industrial chemicals
Mechanism of Action
The mechanism of action of dimethyl 3-methoxyphthalate involves its interaction with specific molecular targets and pathways. The compound can act as a substrate for various enzymes, leading to its conversion into active metabolites. These metabolites may exert their effects by interacting with cellular receptors, enzymes, or other biomolecules, thereby influencing biological processes .
Comparison with Similar Compounds
Dimethyl 3-methoxyphthalate can be compared with other similar compounds, such as:
Dimethyl 4-methoxyphthalate: This compound has a similar structure but with the methoxy group at the 4-position instead of the 3-position.
Dimethyl phthalate: Lacking the methoxy group, this compound has different reactivity and applications compared to this compound.
The uniqueness of this compound lies in its specific substitution pattern, which influences its reactivity and suitability for various applications .
Properties
IUPAC Name |
dimethyl 3-methoxybenzene-1,2-dicarboxylate | |
---|---|---|
Details | Computed by LexiChem 2.6.6 (PubChem release 2019.06.18) | |
Source | PubChem | |
URL | https://pubchem.ncbi.nlm.nih.gov | |
Description | Data deposited in or computed by PubChem | |
InChI |
InChI=1S/C11H12O5/c1-14-8-6-4-5-7(10(12)15-2)9(8)11(13)16-3/h4-6H,1-3H3 | |
Details | Computed by InChI 1.0.5 (PubChem release 2019.06.18) | |
Source | PubChem | |
URL | https://pubchem.ncbi.nlm.nih.gov | |
Description | Data deposited in or computed by PubChem | |
InChI Key |
KKSNRHOMXHCRGF-UHFFFAOYSA-N | |
Details | Computed by InChI 1.0.5 (PubChem release 2019.06.18) | |
Source | PubChem | |
URL | https://pubchem.ncbi.nlm.nih.gov | |
Description | Data deposited in or computed by PubChem | |
Canonical SMILES |
COC1=CC=CC(=C1C(=O)OC)C(=O)OC | |
Details | Computed by OEChem 2.1.5 (PubChem release 2019.06.18) | |
Source | PubChem | |
URL | https://pubchem.ncbi.nlm.nih.gov | |
Description | Data deposited in or computed by PubChem | |
Molecular Formula |
C11H12O5 | |
Details | Computed by PubChem 2.1 (PubChem release 2019.06.18) | |
Source | PubChem | |
URL | https://pubchem.ncbi.nlm.nih.gov | |
Description | Data deposited in or computed by PubChem | |
DSSTOX Substance ID |
DTXSID50311517 | |
Record name | dimethyl 3-methoxyphthalate | |
Source | EPA DSSTox | |
URL | https://comptox.epa.gov/dashboard/DTXSID50311517 | |
Description | DSSTox provides a high quality public chemistry resource for supporting improved predictive toxicology. | |
Molecular Weight |
224.21 g/mol | |
Details | Computed by PubChem 2.1 (PubChem release 2021.05.07) | |
Source | PubChem | |
URL | https://pubchem.ncbi.nlm.nih.gov | |
Description | Data deposited in or computed by PubChem | |
CAS No. |
32136-52-0 | |
Record name | NSC243741 | |
Source | DTP/NCI | |
URL | https://dtp.cancer.gov/dtpstandard/servlet/dwindex?searchtype=NSC&outputformat=html&searchlist=243741 | |
Description | The NCI Development Therapeutics Program (DTP) provides services and resources to the academic and private-sector research communities worldwide to facilitate the discovery and development of new cancer therapeutic agents. | |
Explanation | Unless otherwise indicated, all text within NCI products is free of copyright and may be reused without our permission. Credit the National Cancer Institute as the source. | |
Record name | dimethyl 3-methoxyphthalate | |
Source | EPA DSSTox | |
URL | https://comptox.epa.gov/dashboard/DTXSID50311517 | |
Description | DSSTox provides a high quality public chemistry resource for supporting improved predictive toxicology. | |
Retrosynthesis Analysis
AI-Powered Synthesis Planning: Our tool employs the Template_relevance Pistachio, Template_relevance Bkms_metabolic, Template_relevance Pistachio_ringbreaker, Template_relevance Reaxys, Template_relevance Reaxys_biocatalysis model, leveraging a vast database of chemical reactions to predict feasible synthetic routes.
One-Step Synthesis Focus: Specifically designed for one-step synthesis, it provides concise and direct routes for your target compounds, streamlining the synthesis process.
Accurate Predictions: Utilizing the extensive PISTACHIO, BKMS_METABOLIC, PISTACHIO_RINGBREAKER, REAXYS, REAXYS_BIOCATALYSIS database, our tool offers high-accuracy predictions, reflecting the latest in chemical research and data.
Strategy Settings
Precursor scoring | Relevance Heuristic |
---|---|
Min. plausibility | 0.01 |
Model | Template_relevance |
Template Set | Pistachio/Bkms_metabolic/Pistachio_ringbreaker/Reaxys/Reaxys_biocatalysis |
Top-N result to add to graph | 6 |
Feasible Synthetic Routes
Disclaimer and Information on In-Vitro Research Products
Please be aware that all articles and product information presented on BenchChem are intended solely for informational purposes. The products available for purchase on BenchChem are specifically designed for in-vitro studies, which are conducted outside of living organisms. In-vitro studies, derived from the Latin term "in glass," involve experiments performed in controlled laboratory settings using cells or tissues. It is important to note that these products are not categorized as medicines or drugs, and they have not received approval from the FDA for the prevention, treatment, or cure of any medical condition, ailment, or disease. We must emphasize that any form of bodily introduction of these products into humans or animals is strictly prohibited by law. It is essential to adhere to these guidelines to ensure compliance with legal and ethical standards in research and experimentation.