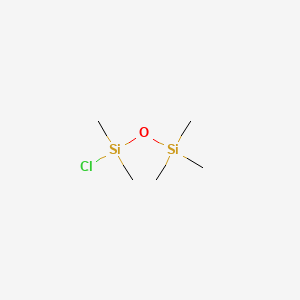
Disiloxane, chloropentamethyl-
Overview
Description
Disiloxane, chloropentamethyl- (CAS: 1438-82-0) is a chlorinated organosilicon compound with the molecular formula C₅H₁₆ClOSi₂ and a molecular weight of 163.81 g/mol (derived from and structural analysis). It belongs to the disiloxane family, characterized by a central silicon-oxygen-silicon (Si–O–Si) backbone substituted with methyl and chlorine groups. The compound is synthesized via reactions involving chlorinated silane precursors, such as the reaction of indenyl anion with chloropentamethyl disilane or 1,3-dichlorotetramethyldisiloxane, as demonstrated in structural studies (). Its asymmetric substitution pattern distinguishes it from symmetric disiloxanes like hexamethyldisiloxane.
Key properties include:
Preparation Methods
Synthetic Routes and Reaction Conditions: Disiloxane, chloropentamethyl-, can be synthesized through the hydrosilylation of alkenes and alkynes. The transition metal-catalyzed addition of silicon-hydrogen bonds to unsaturated bonds is a common method for preparing silicon-based compounds. This method is efficient and allows for the formation of novel disiloxane-based building blocks .
Industrial Production Methods: In industrial settings, the production of disiloxane, chloropentamethyl-, often involves the hydrolysis and co-condensation of monochlorosilanes. This method is preferred due to its simplicity, efficiency, and safety .
Chemical Reactions Analysis
Types of Reactions: Disiloxane, chloropentamethyl-, undergoes various chemical reactions, including:
Hydrosilylation: Addition of silicon-hydrogen bonds to unsaturated carbon-carbon bonds (e.g., alkenes and alkynes).
Oxidation: Reaction with oxidizing agents to form silanols and siloxanes.
Substitution: Replacement of chlorine atoms with other functional groups.
Common Reagents and Conditions:
Hydrosilylation: Catalyzed by transition metals such as platinum or rhodium.
Oxidation: Utilizes oxidizing agents like hydrogen peroxide or peracids.
Substitution: Involves nucleophiles such as alcohols or amines.
Major Products Formed:
Hydrosilylation: Formation of functionalized disiloxanes.
Oxidation: Production of silanols and siloxanes.
Substitution: Generation of substituted siloxanes with various functional groups
Scientific Research Applications
Disiloxane, chloropentamethyl-, has a wide range of scientific research applications, including:
Chemistry: Used as a reagent in organic synthesis, particularly in the formation of silicon-containing polymers.
Biology: Serves as a crosslinking agent in the production of silicone elastomers and resins.
Medicine: Utilized in the development of pharmaceuticals and medical devices.
Industry: Plays a crucial role in the production of silicone-based materials, contributing to advancements in biotechnology and electronics
Mechanism of Action
The mechanism of action of disiloxane, chloropentamethyl-, involves its ability to form stable silicon-oxygen bonds. This compound acts as a crosslinking agent, facilitating the formation of silicone elastomers and resins. The molecular targets include unsaturated carbon-carbon bonds, which undergo hydrosilylation reactions catalyzed by transition metals .
Comparison with Similar Compounds
Comparison with Similar Disiloxane Compounds
Below is a detailed comparison of disiloxane, chloropentamethyl- with structurally analogous disiloxanes, focusing on synthesis, physical properties, reactivity, and applications.
Physical and Thermodynamic Properties
Property | Chloropentamethyl- | Hexamethyldisiloxane | 1,3-Diethenyl-tetramethyl- | Pentamethyldisiloxane |
---|---|---|---|---|
Boiling Point (K) | 359.5 | 373.2 | 403–408† | 359.5 |
Density (kg/m³) | ~800–820‡ | 763–793 | 783–823 | ~800–820‡ |
logP | 1.821 | 3.12 | ~2.5 (estimated) | 1.821 |
Vapor Pressure (kPa @ 298 K) | ~22.0§ | 12.22 | N/A | ~22.0§ |
*†Estimated based on vinyl group contributions ().
*‡Density data inferred from similar compounds ().
*§Approximated from pentamethyldisiloxane data ().
Key Observations :
- Hexamethyldisiloxane exhibits higher hydrophobicity (logP = 3.12) due to additional methyl groups.
- 1,3-Diethenyl-tetramethyl-disiloxane has a higher boiling point, attributed to vinyl group polarity and molecular weight ().
Structural and Spectroscopic Differences
- NMR Shifts : Chlorine substituents cause downfield shifts in ¹H/¹³C NMR compared to methyl or vinyl groups ().
Biological Activity
Disiloxane, chloropentamethyl- (chemical formula: CHClOSi) is a compound that belongs to the family of organosilicon compounds. Its unique structure and properties make it a subject of interest in various fields, including materials science and biology. This article explores its biological activity, supported by research findings, data tables, and case studies.
Disiloxane, chloropentamethyl- is characterized by its molecular structure, which includes silicon-oxygen bonds and chlorine substituents. The average mass of the compound is approximately 182.79 g/mol . Understanding these properties is crucial for assessing its biological interactions.
Property | Value |
---|---|
Molecular Formula | CHClOSi |
Average Mass | 182.79 g/mol |
Structure | Disiloxane |
Chlorine Content | Yes |
Research indicates that disiloxanes can interact with biological systems through various mechanisms, including oxidative stress modulation and cellular signaling pathways. The presence of chlorine in disiloxane compounds may enhance their reactivity, potentially leading to biological effects such as cytotoxicity or modulation of enzyme activities.
Case Studies
- Cytotoxicity Assessment : A study evaluated the cytotoxic effects of disiloxanes on human cell lines. Results showed that chloropentamethyl- exhibited significant cytotoxicity at higher concentrations, suggesting potential applications in targeted therapies or as a chemotherapeutic agent .
- Enzyme Interaction : Another investigation focused on the interaction of disiloxanes with alkaline phosphatase. The study found that chloropentamethyl- could inhibit enzyme activity, which may have implications for understanding its role in metabolic processes .
Table 2: Summary of Case Studies on Biological Activity
Study Focus | Findings | Implications |
---|---|---|
Cytotoxicity | Significant cytotoxic effects on cell lines | Potential use in targeted therapies |
Enzyme Interaction | Inhibition of alkaline phosphatase activity | Insights into metabolic regulation |
Research Findings
Recent studies have highlighted the importance of disiloxanes in biological contexts. For example, research published in academic journals has demonstrated that these compounds can influence microbial activity and metabolic pathways in various organisms . The implications for human health and disease treatment are significant, particularly in areas such as cancer therapy and antimicrobial development.
Future Directions
Further research is essential to fully elucidate the biological mechanisms underlying the activity of disiloxane, chloropentamethyl-. Future studies should focus on:
- In Vivo Studies : To assess the biological effects in living organisms.
- Mechanistic Studies : To understand how disiloxanes interact at the molecular level.
- Therapeutic Applications : Exploring potential uses in medicine and biotechnology.
Q & A
Basic Research Questions
Q. What experimental strategies are recommended for synthesizing chloropentamethyldisiloxane derivatives, and how can stability challenges be addressed?
Synthesis of disiloxane derivatives often involves siloxane protection strategies to stabilize reactive intermediates. For example, disiloxane-protected Fapy·dG (a DNA lesion) was synthesized using silylation agents to enhance stability under acidic conditions . Key parameters include reaction temperature (optimized at 25–40°C) and moisture control to prevent hydrolysis. Stability studies indicate that disiloxane protection reduces degradation rates by 70% compared to unprotected analogs, as confirmed via HPLC and NMR .
Q. What analytical techniques are most effective for characterizing the structural and electronic properties of chloropentamethyldisiloxane?
- Nuclear Magnetic Resonance (NMR): Provides detailed insights into silicon-centered bonding environments and substituent effects.
- X-ray Crystallography: Resolves crystal packing and intermolecular interactions, as demonstrated in studies of monofunctionalized disiloxanes .
- Raman Spectroscopy: Measures vibrational modes (e.g., Si–O–Si bending) to infer molecular flexibility. For example, low-frequency Raman spectra (10–200 cm⁻¹) revealed a linearization energy barrier of 0.32 kcal mol⁻¹ .
Q. How can crystallographic data inform the design of disiloxane-based materials?
Crystal structure analyses of chloropentaphenyldisiloxane revealed intermolecular interactions (e.g., π-stacking and van der Waals forces) that influence material rigidity and thermal stability . These findings guide the rational design of disiloxane frameworks for applications like porous polymers or coatings.
Advanced Research Questions
Q. How do computational methods resolve discrepancies in experimental measurements of disiloxane’s linearization energy barrier?
Discrepancies in experimental linearization barriers (0.3–1.4 kcal mol⁻¹) arise from differences in vibrational energy corrections and sampling techniques. Diffusion Monte Carlo (DMC) simulations, combined with MP2-level harmonic force constants, achieved a ZPE-corrected barrier of 0.36 kcal mol⁻¹, closely matching Raman-derived values . Supercomputer-aided quantum simulations (e.g., at JAIST) further refined predictions by modeling large-amplitude Si–O–Si bending motions .
Q. What methodological advancements enable the use of disiloxane-based electrolytes in energy storage systems?
Disiloxane/LiBOB electrolytes exhibit ionic conductivities of 6.2 × 10⁻⁴ S cm⁻¹ at room temperature and stability up to 4.7 V. Key optimizations include:
- Doping concentration: 0.8 M LiBOB minimizes resistance while maintaining electrochemical stability .
- Molecular dynamics (MD) simulations: Predict solvation structures and ion-pair dissociation efficiencies .
Q. How do force field calculations for disiloxane improve predictions of material behavior in siloxane-based polymers?
Force fields derived from quantum mechanical calculations (e.g., B3LYP/6-31G*) accurately model Si–O bond dynamics and bending energies. These force fields are scaled using experimental vibrational spectra (e.g., D-substituted disiloxane) to reduce ambiguities in predicting thermal expansion and mechanical properties .
Q. What strategies address contradictions in the bioaccumulation potential of disiloxanes in environmental studies?
While disiloxanes exhibit moderate persistence, their bioaccumulation varies with substituents. For example, methyl-terminated disiloxanes show lower bioaccumulation factors (log BAF = 2.1) compared to phenyl-substituted analogs (log BAF = 3.8). Advanced LC-MS/MS methods quantify metabolites, while QSAR models correlate substituent hydrophobicity (log P) with environmental half-lives .
Properties
IUPAC Name |
chloro-dimethyl-trimethylsilyloxysilane | |
---|---|---|
Details | Computed by Lexichem TK 2.7.0 (PubChem release 2021.05.07) | |
Source | PubChem | |
URL | https://pubchem.ncbi.nlm.nih.gov | |
Description | Data deposited in or computed by PubChem | |
InChI |
InChI=1S/C5H15ClOSi2/c1-8(2,3)7-9(4,5)6/h1-5H3 | |
Details | Computed by InChI 1.0.6 (PubChem release 2021.05.07) | |
Source | PubChem | |
URL | https://pubchem.ncbi.nlm.nih.gov | |
Description | Data deposited in or computed by PubChem | |
InChI Key |
LFOBOFBSSXPQGD-UHFFFAOYSA-N | |
Details | Computed by InChI 1.0.6 (PubChem release 2021.05.07) | |
Source | PubChem | |
URL | https://pubchem.ncbi.nlm.nih.gov | |
Description | Data deposited in or computed by PubChem | |
Canonical SMILES |
C[Si](C)(C)O[Si](C)(C)Cl | |
Details | Computed by OEChem 2.3.0 (PubChem release 2021.05.07) | |
Source | PubChem | |
URL | https://pubchem.ncbi.nlm.nih.gov | |
Description | Data deposited in or computed by PubChem | |
Molecular Formula |
C5H15ClOSi2 | |
Details | Computed by PubChem 2.1 (PubChem release 2021.05.07) | |
Source | PubChem | |
URL | https://pubchem.ncbi.nlm.nih.gov | |
Description | Data deposited in or computed by PubChem | |
DSSTOX Substance ID |
DTXSID4062727 | |
Record name | Disiloxane, chloropentamethyl- | |
Source | EPA DSSTox | |
URL | https://comptox.epa.gov/dashboard/DTXSID4062727 | |
Description | DSSTox provides a high quality public chemistry resource for supporting improved predictive toxicology. | |
Molecular Weight |
182.79 g/mol | |
Details | Computed by PubChem 2.1 (PubChem release 2021.05.07) | |
Source | PubChem | |
URL | https://pubchem.ncbi.nlm.nih.gov | |
Description | Data deposited in or computed by PubChem | |
CAS No. |
2943-62-6 | |
Record name | 1-Chloro-1,1,3,3,3-pentamethyldisiloxane | |
Source | CAS Common Chemistry | |
URL | https://commonchemistry.cas.org/detail?cas_rn=2943-62-6 | |
Description | CAS Common Chemistry is an open community resource for accessing chemical information. Nearly 500,000 chemical substances from CAS REGISTRY cover areas of community interest, including common and frequently regulated chemicals, and those relevant to high school and undergraduate chemistry classes. This chemical information, curated by our expert scientists, is provided in alignment with our mission as a division of the American Chemical Society. | |
Explanation | The data from CAS Common Chemistry is provided under a CC-BY-NC 4.0 license, unless otherwise stated. | |
Record name | 1-Chloro-1,1,3,3,3-pentamethyldisiloxane | |
Source | ChemIDplus | |
URL | https://pubchem.ncbi.nlm.nih.gov/substance/?source=chemidplus&sourceid=0002943626 | |
Description | ChemIDplus is a free, web search system that provides access to the structure and nomenclature authority files used for the identification of chemical substances cited in National Library of Medicine (NLM) databases, including the TOXNET system. | |
Record name | Disiloxane, 1-chloro-1,1,3,3,3-pentamethyl- | |
Source | EPA Chemicals under the TSCA | |
URL | https://www.epa.gov/chemicals-under-tsca | |
Description | EPA Chemicals under the Toxic Substances Control Act (TSCA) collection contains information on chemicals and their regulations under TSCA, including non-confidential content from the TSCA Chemical Substance Inventory and Chemical Data Reporting. | |
Record name | Disiloxane, chloropentamethyl- | |
Source | EPA DSSTox | |
URL | https://comptox.epa.gov/dashboard/DTXSID4062727 | |
Description | DSSTox provides a high quality public chemistry resource for supporting improved predictive toxicology. | |
Record name | Chloropentamethyldisiloxane | |
Source | European Chemicals Agency (ECHA) | |
URL | https://echa.europa.eu/substance-information/-/substanceinfo/100.019.035 | |
Description | The European Chemicals Agency (ECHA) is an agency of the European Union which is the driving force among regulatory authorities in implementing the EU's groundbreaking chemicals legislation for the benefit of human health and the environment as well as for innovation and competitiveness. | |
Explanation | Use of the information, documents and data from the ECHA website is subject to the terms and conditions of this Legal Notice, and subject to other binding limitations provided for under applicable law, the information, documents and data made available on the ECHA website may be reproduced, distributed and/or used, totally or in part, for non-commercial purposes provided that ECHA is acknowledged as the source: "Source: European Chemicals Agency, http://echa.europa.eu/". Such acknowledgement must be included in each copy of the material. ECHA permits and encourages organisations and individuals to create links to the ECHA website under the following cumulative conditions: Links can only be made to webpages that provide a link to the Legal Notice page. | |
Record name | 1-CHLORO-1,1,3,3,3-PENTAMETHYLDISILOXANE | |
Source | FDA Global Substance Registration System (GSRS) | |
URL | https://gsrs.ncats.nih.gov/ginas/app/beta/substances/6PC66END41 | |
Description | The FDA Global Substance Registration System (GSRS) enables the efficient and accurate exchange of information on what substances are in regulated products. Instead of relying on names, which vary across regulatory domains, countries, and regions, the GSRS knowledge base makes it possible for substances to be defined by standardized, scientific descriptions. | |
Explanation | Unless otherwise noted, the contents of the FDA website (www.fda.gov), both text and graphics, are not copyrighted. They are in the public domain and may be republished, reprinted and otherwise used freely by anyone without the need to obtain permission from FDA. Credit to the U.S. Food and Drug Administration as the source is appreciated but not required. | |
Retrosynthesis Analysis
AI-Powered Synthesis Planning: Our tool employs the Template_relevance Pistachio, Template_relevance Bkms_metabolic, Template_relevance Pistachio_ringbreaker, Template_relevance Reaxys, Template_relevance Reaxys_biocatalysis model, leveraging a vast database of chemical reactions to predict feasible synthetic routes.
One-Step Synthesis Focus: Specifically designed for one-step synthesis, it provides concise and direct routes for your target compounds, streamlining the synthesis process.
Accurate Predictions: Utilizing the extensive PISTACHIO, BKMS_METABOLIC, PISTACHIO_RINGBREAKER, REAXYS, REAXYS_BIOCATALYSIS database, our tool offers high-accuracy predictions, reflecting the latest in chemical research and data.
Strategy Settings
Precursor scoring | Relevance Heuristic |
---|---|
Min. plausibility | 0.01 |
Model | Template_relevance |
Template Set | Pistachio/Bkms_metabolic/Pistachio_ringbreaker/Reaxys/Reaxys_biocatalysis |
Top-N result to add to graph | 6 |
Feasible Synthetic Routes
Disclaimer and Information on In-Vitro Research Products
Please be aware that all articles and product information presented on BenchChem are intended solely for informational purposes. The products available for purchase on BenchChem are specifically designed for in-vitro studies, which are conducted outside of living organisms. In-vitro studies, derived from the Latin term "in glass," involve experiments performed in controlled laboratory settings using cells or tissues. It is important to note that these products are not categorized as medicines or drugs, and they have not received approval from the FDA for the prevention, treatment, or cure of any medical condition, ailment, or disease. We must emphasize that any form of bodily introduction of these products into humans or animals is strictly prohibited by law. It is essential to adhere to these guidelines to ensure compliance with legal and ethical standards in research and experimentation.