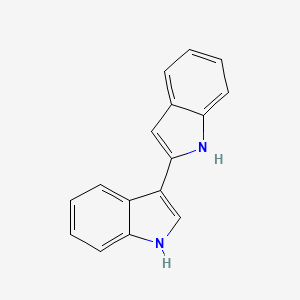
2,3'-Biindole
Overview
Description
2,3'-Biindole (C₁₆H₁₂N₂) is a dimeric indole derivative characterized by two indole moieties connected via a bond between the C2 position of one indole and the C3' position of the other . This structural arrangement distinguishes it from other biindoles, such as 2,2'-biindole and 3,3'-biindole, which exhibit different connectivity patterns. The compound is synthesized through methods like acid-mediated dimerization of indole derivatives, Fischer indole reactions, or double cyclization of 1,3-bis(2-nitrophenyl)-1,3-butadienes under Pd-catalyzed conditions . Notably, only four natural this compound derivatives have been isolated, all featuring an ethylene bridge between the 2 and 3' carbons .
Preparation Methods
Synthetic Routes and Reaction Conditions
One of the common methods for synthesizing 2,3’-biindole involves the homodimerization of indole derivatives. This can be achieved using N-bromosuccinimide (NBS) as a reagent under mild conditions, typically at 15°C. The reaction provides excellent regioselectivity and high efficiency, making it a preferred method for synthesizing 2,3’-biindole .
Another method involves the use of molecular iodine as a catalyst in the presence of sodium bicarbonate. This metal-free approach allows for the formation of C–C and C–N bonds, resulting in the synthesis of 2,3’-biindole at room temperature .
Industrial Production Methods
Industrial production methods for 2,3’-biindole are not extensively documented. the methods mentioned above can be scaled up for industrial applications, given their efficiency and mild reaction conditions.
Chemical Reactions Analysis
Types of Reactions
2,3’-Biindole undergoes various chemical reactions, including:
Oxidation: Oxidative coupling reactions can be used to form biindole derivatives.
Reduction: Reduction reactions can modify the functional groups attached to the indole units.
Substitution: Electrophilic substitution reactions are common, given the electron-rich nature of the indole rings.
Common Reagents and Conditions
Oxidation: Reagents like N-bromosuccinimide (NBS) and molecular iodine are commonly used.
Reduction: Hydrogenation reactions using palladium on carbon (Pd/C) as a catalyst.
Substitution: Electrophilic reagents such as bromine or chlorine can be used under mild conditions.
Major Products Formed
The major products formed from these reactions include various substituted biindole derivatives, which can have different functional groups attached to the indole rings, enhancing their chemical and biological properties .
Scientific Research Applications
Anticancer Potential
Research Findings:
A study published in the Turkish Journal of Biology investigated the antiproliferative and cytotoxic effects of 2,3'-biindole on several cancer cell lines, including C6 (rat brain tumor), HeLa (human cervix carcinoma), and HT29 (human colon carcinoma). The results indicated that this compound significantly inhibited the proliferation of HeLa and HT29 cells compared to the standard drug 5-fluorouracil (5-FU). Notably, it exhibited high cytotoxicity against HeLa cells while showing lower toxicity toward HT29 cells. The compound also inhibited topoisomerase activity without inducing apoptosis, suggesting a unique mechanism of action that warrants further investigation .
Cell Line | Proliferation Inhibition | Cytotoxicity Level |
---|---|---|
C6 | Moderate | High |
HeLa | Significant | High |
HT29 | Significant | Low |
Synthetic Applications
Copper-Catalyzed Reactions:
Recent advancements in synthetic methodologies have employed copper-catalyzed oxidative dearomatization to produce various derivatives of this compound. This method allows for the transformation of readily available indole substrates into complex structures with potential applications in drug discovery. For instance, a study demonstrated the use of copper catalysts to facilitate the formation of spirocyclic indolenines from this compound derivatives, achieving moderate to high yields depending on the substituents present on the phenyl ring .
Reaction Conditions | Yield (%) |
---|---|
Cu(OAc)₂ (10 mol%) | 36 |
Cu(OAc)₂ (20 mol%) | 47 |
Material Science
Organic Semiconductors:
The unique electronic properties of biindoles have led to their exploration as components in organic semiconductors. Research indicates that derivatives of this compound can serve as effective π-conjugated materials due to their inherent stability and electronic characteristics. These materials have potential applications in organic light-emitting diodes (OLEDs) and photovoltaic devices .
Catalytic Applications
Nanocatalysis:
Innovative approaches using heterogeneous nanocatalysts have been developed to synthesize derivatives of this compound efficiently. For example, Fe₃O₄@MCC nanoparticles have been utilized to accelerate multi-component reactions (MCRs), leading to the formation of various biindole derivatives under mild conditions. This method enhances reaction efficiency and product yield, making it a valuable tool for drug discovery and material synthesis .
Mechanism of Action
The mechanism of action of 2,3’-biindole involves its interaction with various molecular targets. It has been shown to inhibit the proliferation of cancer cells by interfering with topoisomerase activity, which is essential for DNA replication and cell division. This inhibition leads to the accumulation of supercoiled DNA, ultimately causing cell death .
Comparison with Similar Compounds
Comparison with Similar Biindoles
2,3'-Biindole vs. 2,2'-Biindole
- Structure : 2,2'-Biindole has a C2–C2' linkage, creating a symmetrical structure, whereas this compound is asymmetric due to its C2–C3' bond .
- Synthesis :
- Natural Occurrence : 2,2'-Biindoles are more common in natural products compared to 2,3'-biindoles, which are rare .
This compound vs. 3,3'-Biindole
- Structure : 3,3'-Biindole features a C3–C3' linkage, resulting in a planar structure conducive to π-π stacking interactions.
- Synthesis: 3,3'-Biindole is synthesized via Pd-catalyzed homocoupling of 1-(2-nitrophenyl)ethenyltin derivatives or Masuda indole borylation-Suzuki arylation sequences . Yields for 3,3'-biindoles range from 64–100% under optimized CuCl/Cu(NO₃)₂ conditions .
Physicochemical Properties
*Synthetic yields vary based on catalytic systems and substrates.
Anticancer Activity
- This compound : Exhibits significant antiproliferative effects against HeLa (IC₅₀ = 18 μM) and HT29 (IC₅₀ = 22 μM) cancer cells, surpassing 5-fluorouracil in potency . Its mechanism involves topoisomerase inhibition rather than apoptosis induction .
- 3,3'-Biindole: Limited anticancer data, but halogenated derivatives from marine sources show cytotoxicity against leukemia cells .
- Indirubin (a biindole dione) : A related compound with antitumor activity, but distinct due to its 2,3-dione structure .
Key Research Findings
Synthetic Advancements: Asymmetric this compound derivatives are synthesized via Brønsted acid-catalyzed C3-arylation of 2-indolylmethanols, enabling access to bioactive scaffolds .
Natural Product Rarity: Only four natural 2,3'-biindoles are known, underscoring the challenges in their isolation and synthesis .
Biological Activity
2,3'-Biindole is a compound that has garnered attention in the field of medicinal chemistry due to its diverse biological activities. This article explores the synthesis, biological properties, and potential therapeutic applications of this compound and its derivatives.
Chemical Structure and Synthesis
This compound is characterized by its unique indole framework, which consists of two indole units linked at the 2 and 3 positions. The synthesis of this compound can be achieved through various methods, including:
- Copper-mediated reactions : A notable method involves the copper-mediated 2,3-difunctionalization of indoles to yield halogenated derivatives of biindoles. This approach utilizes readily available feedstocks and environmentally friendly catalysts, making it a sustainable option for synthesizing biindoles .
- Dynamic kinetic resolution : Another synthetic route employs chiral phosphoric acid-catalyzed dynamic kinetic resolution to produce bisindoles with axial and central chirality .
Anticancer Properties
This compound and its derivatives have shown significant antiproliferative activity against various cancer cell lines. For instance:
- Cytotoxicity Studies : In research involving human prostate cancer cell line DU145, specific derivatives of this compound demonstrated a marked reduction in cell viability. Compounds such as 1-ethyl-3-hydroxy-1,1',3,3'-tetrahydro-2H,2'H-3,3'-biindole-2,2'-dione exhibited IC50 values that indicate potent cytotoxic effects .
- Mechanisms of Action : The mechanisms underlying these anticancer effects include the induction of apoptosis and cell cycle arrest. For example, compound 1e caused an increase in sub-G1 phase cells and apoptosis indicators such as tail DNA formation in comet assays .
Compound | Cell Line | IC50 (μM) | Mechanism |
---|---|---|---|
1e | DU145 | 55 | Apoptosis induction |
1f | DU145 | Similar to 1e | Apoptosis induction |
Other Biological Activities
Beyond anticancer effects, biindoles have been studied for their interactions with various biological targets:
- Cyclin-dependent Kinase Inhibition : Certain biindole derivatives have been identified as potential inhibitors of cyclin-dependent kinases (CDKs), which play crucial roles in cell cycle regulation. For instance, studies indicated that compounds derived from biindole scaffolds could bind to CDK2 with promising inhibitory activity .
- Neuroprotective Effects : Research has also suggested that some biindole derivatives may possess neuroprotective properties by modulating pathways involved in neurodegenerative diseases.
Case Studies
Several case studies highlight the therapeutic potential of this compound:
- Prostate Cancer Treatment : A study demonstrated that treatment with specific biindole derivatives led to significant apoptosis in DU145 cells after prolonged exposure. The morphological changes observed via SEM further corroborated the apoptotic nature of these compounds .
- Targeting CDK Pathways : In another study focused on CDK inhibition, researchers utilized a decorated biindole scaffold to explore its binding affinity and inhibitory effects on CDK activity. This approach provided insights into the structure-activity relationship crucial for developing effective CDK inhibitors .
Q & A
Basic Research Questions
Q. What are the key considerations for synthesizing 2,3'-Biindole with high purity?
- Methodological Answer : Follow established protocols for indole dimerization, such as oxidative coupling or transition-metal-catalyzed cross-coupling reactions. Ensure precise control of reaction conditions (e.g., temperature, solvent, stoichiometry) to minimize byproducts. Characterize intermediates and final products using NMR (e.g., H, C), IR, and mass spectrometry to confirm structural identity and purity (>95%) . For reproducibility, document deviations from literature procedures and validate purity via HPLC .
Q. How should researchers characterize this compound’s structural and electronic properties?
- Methodological Answer : Combine spectroscopic techniques (NMR, UV-Vis) with computational methods (DFT calculations) to analyze electronic transitions and substituent effects. For crystallographic studies, grow single crystals via slow evaporation in non-polar solvents and compare bond lengths/angles with literature data. Note discrepancies in spectral data (e.g., chemical shifts in H NMR) that may arise from solvent polarity or tautomerism .
Q. What analytical methods are most reliable for quantifying this compound in complex matrices?
- Methodological Answer : Use reverse-phase HPLC with UV detection (λ = 280–300 nm) for separation and quantification. Validate the method using spiked samples to assess recovery rates (≥90%) and limit of detection (LOD < 0.1 µg/mL). Cross-check with LC-MS for confirmatory analysis, ensuring ion suppression effects are minimized .
Advanced Research Questions
Q. How can researchers resolve contradictions in spectral data for this compound derivatives?
- Methodological Answer : Investigate potential sources of variability:
- Sample purity : Re-crystallize or use column chromatography to remove impurities.
- Tautomeric equilibria : Conduct variable-temperature NMR to detect dynamic processes.
- Solvent effects : Compare spectra in deuterated solvents with differing polarities (e.g., CDCl₃ vs. DMSO-d₆).
- Instrument calibration : Validate spectrometer settings using internal standards (e.g., TMS) .
- Example : Inconsistent C NMR signals may arise from slow conformational changes; use 2D NMR (HSQC, HMBC) to assign ambiguous peaks .
Q. What strategies are effective for studying structure-activity relationships (SAR) of this compound in biological systems?
- Methodological Answer :
- Systematic substitution : Introduce functional groups (e.g., halogens, methyl) at specific positions and assess biological activity (e.g., IC₅₀ in enzyme assays).
- Molecular docking : Use X-ray or cryo-EM structures of target proteins to predict binding modes.
- Pharmacokinetic profiling : Measure solubility, logP, and metabolic stability via in vitro assays (e.g., microsomal stability tests) .
Q. How should researchers address conflicting results between computational predictions and experimental data for this compound reactivity?
- Methodological Answer :
- Validate computational models : Compare multiple DFT functionals (e.g., B3LYP vs. M06-2X) and basis sets.
- Experimental replication : Repeat reactions under inert atmospheres to exclude oxidation artifacts.
- Error analysis : Quantify uncertainties in computational energy barriers (±2–3 kcal/mol) and correlate with kinetic data (e.g., Arrhenius plots) .
Q. What experimental designs are optimal for probing this compound’s role in multi-step catalytic cycles?
- Methodological Answer :
- Kinetic studies : Use stopped-flow spectroscopy or quenching experiments to identify rate-determining steps.
- Isotopic labeling : Incorporate N or C isotopes to track mechanistic pathways via NMR or MS.
- In situ monitoring : Employ operando techniques (e.g., Raman spectroscopy) to detect transient intermediates .
Q. Data Analysis and Contradiction Management
Q. How to statistically analyze discrepancies in biological activity data for this compound analogs?
- Methodological Answer :
- Outlier detection : Apply Grubbs’ test or Dixon’s Q-test to exclude anomalous data points.
- Multivariate analysis : Use PCA or clustering algorithms to identify structural features correlating with activity.
- Reproducibility checks : Replicate assays across independent labs and perform meta-analysis to assess inter-lab variability .
Q. What frameworks guide the prioritization of contradictory hypotheses in this compound research?
- Methodological Answer : Apply the FINER criteria (Feasible, Interesting, Novel, Ethical, Relevant) to rank hypotheses. For example:
- Prioritize studies resolving tautomerism’s impact on reactivity over incremental synthetic optimizations.
- Use the PICO framework to define populations (e.g., catalytic systems), interventions (e.g., substituent effects), and outcomes (e.g., yield improvements) .
Q. Ethical and Reproducibility Considerations
Q. What steps ensure ethical reporting of this compound research?
- Methodological Answer :
- Data transparency : Archive raw spectra, chromatograms, and crystallographic data in public repositories (e.g., Cambridge Structural Database).
- Citation rigor : Cite primary sources for synthetic protocols, avoiding non-peer-reviewed platforms (e.g., commercial websites) .
- Conflict of interest : Disclose funding sources or intellectual property ties in publications .
Properties
IUPAC Name |
2-(1H-indol-3-yl)-1H-indole | |
---|---|---|
Details | Computed by Lexichem TK 2.7.0 (PubChem release 2021.05.07) | |
Source | PubChem | |
URL | https://pubchem.ncbi.nlm.nih.gov | |
Description | Data deposited in or computed by PubChem | |
InChI |
InChI=1S/C16H12N2/c1-3-7-14-11(5-1)9-16(18-14)13-10-17-15-8-4-2-6-12(13)15/h1-10,17-18H | |
Details | Computed by InChI 1.0.6 (PubChem release 2021.05.07) | |
Source | PubChem | |
URL | https://pubchem.ncbi.nlm.nih.gov | |
Description | Data deposited in or computed by PubChem | |
InChI Key |
KWGGLCJWTVNIAO-UHFFFAOYSA-N | |
Details | Computed by InChI 1.0.6 (PubChem release 2021.05.07) | |
Source | PubChem | |
URL | https://pubchem.ncbi.nlm.nih.gov | |
Description | Data deposited in or computed by PubChem | |
Canonical SMILES |
C1=CC=C2C(=C1)C=C(N2)C3=CNC4=CC=CC=C43 | |
Details | Computed by OEChem 2.3.0 (PubChem release 2021.05.07) | |
Source | PubChem | |
URL | https://pubchem.ncbi.nlm.nih.gov | |
Description | Data deposited in or computed by PubChem | |
Molecular Formula |
C16H12N2 | |
Details | Computed by PubChem 2.1 (PubChem release 2021.05.07) | |
Source | PubChem | |
URL | https://pubchem.ncbi.nlm.nih.gov | |
Description | Data deposited in or computed by PubChem | |
DSSTOX Substance ID |
DTXSID60295753 | |
Record name | 2,3'-Biindole | |
Source | EPA DSSTox | |
URL | https://comptox.epa.gov/dashboard/DTXSID60295753 | |
Description | DSSTox provides a high quality public chemistry resource for supporting improved predictive toxicology. | |
Molecular Weight |
232.28 g/mol | |
Details | Computed by PubChem 2.1 (PubChem release 2021.05.07) | |
Source | PubChem | |
URL | https://pubchem.ncbi.nlm.nih.gov | |
Description | Data deposited in or computed by PubChem | |
CAS No. |
27393-85-7 | |
Record name | 2,3'-Biindole | |
Source | DTP/NCI | |
URL | https://dtp.cancer.gov/dtpstandard/servlet/dwindex?searchtype=NSC&outputformat=html&searchlist=105330 | |
Description | The NCI Development Therapeutics Program (DTP) provides services and resources to the academic and private-sector research communities worldwide to facilitate the discovery and development of new cancer therapeutic agents. | |
Explanation | Unless otherwise indicated, all text within NCI products is free of copyright and may be reused without our permission. Credit the National Cancer Institute as the source. | |
Record name | 2,3'-Biindole | |
Source | EPA DSSTox | |
URL | https://comptox.epa.gov/dashboard/DTXSID60295753 | |
Description | DSSTox provides a high quality public chemistry resource for supporting improved predictive toxicology. | |
Retrosynthesis Analysis
AI-Powered Synthesis Planning: Our tool employs the Template_relevance Pistachio, Template_relevance Bkms_metabolic, Template_relevance Pistachio_ringbreaker, Template_relevance Reaxys, Template_relevance Reaxys_biocatalysis model, leveraging a vast database of chemical reactions to predict feasible synthetic routes.
One-Step Synthesis Focus: Specifically designed for one-step synthesis, it provides concise and direct routes for your target compounds, streamlining the synthesis process.
Accurate Predictions: Utilizing the extensive PISTACHIO, BKMS_METABOLIC, PISTACHIO_RINGBREAKER, REAXYS, REAXYS_BIOCATALYSIS database, our tool offers high-accuracy predictions, reflecting the latest in chemical research and data.
Strategy Settings
Precursor scoring | Relevance Heuristic |
---|---|
Min. plausibility | 0.01 |
Model | Template_relevance |
Template Set | Pistachio/Bkms_metabolic/Pistachio_ringbreaker/Reaxys/Reaxys_biocatalysis |
Top-N result to add to graph | 6 |
Feasible Synthetic Routes
Disclaimer and Information on In-Vitro Research Products
Please be aware that all articles and product information presented on BenchChem are intended solely for informational purposes. The products available for purchase on BenchChem are specifically designed for in-vitro studies, which are conducted outside of living organisms. In-vitro studies, derived from the Latin term "in glass," involve experiments performed in controlled laboratory settings using cells or tissues. It is important to note that these products are not categorized as medicines or drugs, and they have not received approval from the FDA for the prevention, treatment, or cure of any medical condition, ailment, or disease. We must emphasize that any form of bodily introduction of these products into humans or animals is strictly prohibited by law. It is essential to adhere to these guidelines to ensure compliance with legal and ethical standards in research and experimentation.