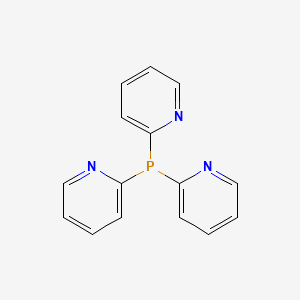
Tris(2-pyridyl)phosphine
Overview
Description
Tris(2-pyridyl)phosphine is a versatile ligand that has garnered significant interest in coordination chemistry, catalysis, and material science. The compound features a phosphorus atom bonded to three 2-pyridyl groups, combining both “soft” (phosphorus) and “hard” (nitrogen) donor sites. This unique combination makes it an important ligand for designing catalysts, luminescent materials, and potential drugs .
Mechanism of Action
Target of Action
Tris(2-pyridyl)phosphine primarily targets pnictogen acceptors, such as arsenic and antimony . These elements play a crucial role in the formation of cationic complexes when interacting with the this compound ligand .
Mode of Action
The compound interacts with its targets through a process known as chloride ion abstraction. In the presence of the ligand, this process involves the use of TMSOTf to abstract chloride ions from AsCl3 . This results in the formation of [P(Pyr)3As][OTf]3, a trication that adopts a C3v symmetric cage structure . The reaction proceeds via the intermediate [P(Pyr)3AsCl][OTf]2, which undergoes chloride exchange to give [P(Pyr)3As][OTf]3 and [P(Pyr)3AsCl2][OTf] .
Biochemical Pathways
The compound’s ability to form cationic complexes with arsenic and antimony suggests that it may influence pathways involving these elements .
Result of Action
The interaction of this compound with its targets results in the formation of cationic complexes . These complexes have a C3v symmetric cage structure , indicating a significant change in the molecular configuration of the targets.
Action Environment
The action of this compound can be influenced by environmental factors. For instance, the compound’s ability to form cationic complexes is facilitated by the presence of TMSOTf . Additionally, the compound’s reaction with 2-bromopyridine and red phosphorus under microwave irradiation in a superbasic KOH/DMSO (H2O) suspension yields this compound . This suggests that the compound’s action, efficacy, and stability can be influenced by factors such as temperature, pH, and the presence of other chemical agents.
Biochemical Analysis
Biochemical Properties
Tris(2-pyridyl)phosphine has been found to interact with various enzymes, proteins, and other biomolecules . The nature of these interactions is complex and multifaceted, involving a range of biochemical reactions .
Cellular Effects
This compound has been observed to have significant effects on various types of cells and cellular processes . It influences cell function, including impacts on cell signaling pathways, gene expression, and cellular metabolism .
Molecular Mechanism
The mechanism of action of this compound is intricate. It exerts its effects at the molecular level, including binding interactions with biomolecules, enzyme inhibition or activation, and changes in gene expression .
Temporal Effects in Laboratory Settings
In laboratory settings, the effects of this compound have been observed to change over time . This includes information on the product’s stability, degradation, and any long-term effects on cellular function observed in in vitro or in vivo studies .
Dosage Effects in Animal Models
The effects of this compound vary with different dosages in animal models . This includes any threshold effects observed in these studies, as well as any toxic or adverse effects at high doses .
Metabolic Pathways
This compound is involved in several metabolic pathways . This includes interactions with various enzymes or cofactors, and effects on metabolic flux or metabolite levels .
Transport and Distribution
This compound is transported and distributed within cells and tissues in a specific manner . This includes interactions with any transporters or binding proteins, as well as effects on its localization or accumulation .
Subcellular Localization
The subcellular localization of this compound and its effects on activity or function are of significant interest . This includes any targeting signals or post-translational modifications that direct it to specific compartments or organelles .
Preparation Methods
Synthetic Routes and Reaction Conditions: Tris(2-pyridyl)phosphine can be synthesized through various methods. One common approach involves the reaction of 2-pyridylmagnesium bromide with phosphorus trichloride. The reaction typically occurs in an inert atmosphere, such as nitrogen or argon, and requires careful control of temperature and stoichiometry to ensure high yields .
Industrial Production Methods: While specific industrial production methods for this compound are not widely documented, the general principles of organophosphorus chemistry apply. Industrial synthesis would likely involve scalable reactions with optimized conditions for yield and purity, utilizing automated systems for reagent addition and product isolation .
Chemical Reactions Analysis
Types of Reactions: Tris(2-pyridyl)phosphine undergoes various chemical reactions, including:
Oxidation: The compound can be oxidized to form this compound oxide.
Substitution: It can participate in ligand substitution reactions, where it replaces other ligands in metal complexes.
Common Reagents and Conditions:
Oxidation: Common oxidizing agents include hydrogen peroxide and oxygen.
Substitution: Reactions often involve metal salts such as copper(II) hexafluoroacetylacetonate.
Major Products:
Oxidation: this compound oxide.
Substitution: Various metal complexes, depending on the metal salt used.
Scientific Research Applications
Tris(2-pyridyl)phosphine has a wide range of applications in scientific research:
Chemistry: It is used as a ligand in coordination chemistry to form stable metal complexes.
Biology: The compound’s metal complexes are studied for their potential biological activities.
Medicine: Research is ongoing into its potential use in drug design and delivery.
Industry: It is used in catalysis for various industrial processes, including polymerization and hydrogenation reactions
Comparison with Similar Compounds
- Tris(2-pyridyl)phosphine oxide
- Tris(2-pyridyl)ethylphosphine
- Tris(2-pyridyl)ethylphosphine oxide
Uniqueness: this compound is unique due to its combination of “soft” and “hard” donor sites, which allows it to form stable complexes with a wide range of metal ions. This versatility makes it particularly valuable in catalysis and material science .
Properties
IUPAC Name |
tripyridin-2-ylphosphane | |
---|---|---|
Details | Computed by Lexichem TK 2.7.0 (PubChem release 2021.05.07) | |
Source | PubChem | |
URL | https://pubchem.ncbi.nlm.nih.gov | |
Description | Data deposited in or computed by PubChem | |
InChI |
InChI=1S/C15H12N3P/c1-4-10-16-13(7-1)19(14-8-2-5-11-17-14)15-9-3-6-12-18-15/h1-12H | |
Details | Computed by InChI 1.0.6 (PubChem release 2021.05.07) | |
Source | PubChem | |
URL | https://pubchem.ncbi.nlm.nih.gov | |
Description | Data deposited in or computed by PubChem | |
InChI Key |
LDSMXGPRCFQXSK-UHFFFAOYSA-N | |
Details | Computed by InChI 1.0.6 (PubChem release 2021.05.07) | |
Source | PubChem | |
URL | https://pubchem.ncbi.nlm.nih.gov | |
Description | Data deposited in or computed by PubChem | |
Canonical SMILES |
C1=CC=NC(=C1)P(C2=CC=CC=N2)C3=CC=CC=N3 | |
Details | Computed by OEChem 2.3.0 (PubChem release 2021.05.07) | |
Source | PubChem | |
URL | https://pubchem.ncbi.nlm.nih.gov | |
Description | Data deposited in or computed by PubChem | |
Molecular Formula |
C15H12N3P | |
Details | Computed by PubChem 2.1 (PubChem release 2021.05.07) | |
Source | PubChem | |
URL | https://pubchem.ncbi.nlm.nih.gov | |
Description | Data deposited in or computed by PubChem | |
DSSTOX Substance ID |
DTXSID30453969 | |
Record name | Pyridine, 2,2',2''-phosphinidynetris- | |
Source | EPA DSSTox | |
URL | https://comptox.epa.gov/dashboard/DTXSID30453969 | |
Description | DSSTox provides a high quality public chemistry resource for supporting improved predictive toxicology. | |
Molecular Weight |
265.25 g/mol | |
Details | Computed by PubChem 2.1 (PubChem release 2021.05.07) | |
Source | PubChem | |
URL | https://pubchem.ncbi.nlm.nih.gov | |
Description | Data deposited in or computed by PubChem | |
CAS No. |
26437-48-9 | |
Record name | Pyridine, 2,2',2''-phosphinidynetris- | |
Source | EPA DSSTox | |
URL | https://comptox.epa.gov/dashboard/DTXSID30453969 | |
Description | DSSTox provides a high quality public chemistry resource for supporting improved predictive toxicology. | |
Record name | 2,2',2''-Phosphinetriyltripyridine | |
Source | European Chemicals Agency (ECHA) | |
URL | https://echa.europa.eu/information-on-chemicals | |
Description | The European Chemicals Agency (ECHA) is an agency of the European Union which is the driving force among regulatory authorities in implementing the EU's groundbreaking chemicals legislation for the benefit of human health and the environment as well as for innovation and competitiveness. | |
Explanation | Use of the information, documents and data from the ECHA website is subject to the terms and conditions of this Legal Notice, and subject to other binding limitations provided for under applicable law, the information, documents and data made available on the ECHA website may be reproduced, distributed and/or used, totally or in part, for non-commercial purposes provided that ECHA is acknowledged as the source: "Source: European Chemicals Agency, http://echa.europa.eu/". Such acknowledgement must be included in each copy of the material. ECHA permits and encourages organisations and individuals to create links to the ECHA website under the following cumulative conditions: Links can only be made to webpages that provide a link to the Legal Notice page. | |
Retrosynthesis Analysis
AI-Powered Synthesis Planning: Our tool employs the Template_relevance Pistachio, Template_relevance Bkms_metabolic, Template_relevance Pistachio_ringbreaker, Template_relevance Reaxys, Template_relevance Reaxys_biocatalysis model, leveraging a vast database of chemical reactions to predict feasible synthetic routes.
One-Step Synthesis Focus: Specifically designed for one-step synthesis, it provides concise and direct routes for your target compounds, streamlining the synthesis process.
Accurate Predictions: Utilizing the extensive PISTACHIO, BKMS_METABOLIC, PISTACHIO_RINGBREAKER, REAXYS, REAXYS_BIOCATALYSIS database, our tool offers high-accuracy predictions, reflecting the latest in chemical research and data.
Strategy Settings
Precursor scoring | Relevance Heuristic |
---|---|
Min. plausibility | 0.01 |
Model | Template_relevance |
Template Set | Pistachio/Bkms_metabolic/Pistachio_ringbreaker/Reaxys/Reaxys_biocatalysis |
Top-N result to add to graph | 6 |
Feasible Synthetic Routes
Q1: What is the molecular formula and weight of tris(2-pyridyl)phosphine?
A1: this compound has a molecular formula of C₁₅H₁₂N₃P and a molecular weight of 265.3 g/mol. []
Q2: How does the structure of this compound influence its coordination behavior?
A2: this compound features a central phosphorus atom bonded to three 2-pyridyl groups. This arrangement creates a "scorpionate" ligand, capable of both P-monodentate and P,N,N'-tridentate coordination modes depending on the metal center and reaction conditions. [, , , , , ]
Q3: What spectroscopic techniques are commonly used to characterize this compound and its complexes?
A3: Common techniques include:
- NMR spectroscopy: ¹H, ¹³C{¹H}, and ³¹P{¹H} NMR are invaluable for structural elucidation, especially for characterizing isomers and dynamic processes in solution. []
- IR Spectroscopy: Provides information about the presence of functional groups, particularly useful for analyzing carbonyl complexes and studying metal-ligand interactions. [, , , ]
- X-ray crystallography: Used to determine the solid-state structures of this compound complexes, offering insights into coordination geometries and intermolecular interactions. [, , , , , , ]
Q4: How does the phosphorus lone pair in this compound contribute to its reactivity?
A4: The phosphorus lone pair in this compound makes it a strong σ-donor ligand, readily forming complexes with transition metals. DFT calculations suggest that despite the formal +3 charge in some complexes like [P(Pyr)₃As]³⁺, the phosphorus lone pair retains significant electron density, indicating potential nucleophilic behavior. [, ]
Q5: What are some examples of metal complexes formed with this compound?
A5: this compound forms complexes with a wide range of transition metals, including Ru, Rh, Cu, Au, Ag, Mn, Co, Ni, Mo, W, Re, and Tc. These complexes vary in their structures and properties, showcasing the versatility of this ligand. [, , , , , , , , , , , , , , , , ]
Q6: How does this compound facilitate P-C bond cleavage reactions?
A6: Research suggests that the coordination of this compound to metal centers like ruthenium can activate P-C bonds, particularly P-aryl and P-pyridyl bonds, leading to their cleavage under mild conditions. The exact mechanism remains to be fully elucidated. [, ]
Q7: What catalytic applications of this compound complexes have been explored?
A7: Notable examples include: * Hydroformylation: Rhodium complexes with this compound have shown activity in the hydroformylation of alkenes, converting them to aldehydes. [] * Carbonylation: Palladium complexes with this compound have demonstrated catalytic activity in the carbonylation of alcohols, illustrating potential for applications in organic synthesis. [] * Water oxidation: Ruthenium complexes of this compound oxide exhibit rapid water oxidation electrocatalysis, highlighting potential in sustainable energy research. [] * Ethylene Polymerization: Iron(II) and cobalt(II) complexes with this compound have been studied for their potential as catalysts in ethylene polymerization. []
Q8: What are some interesting reactions of this compound with organic molecules?
A8: this compound exhibits intriguing reactivity with certain organic molecules, leading to the formation of unique heterocyclic compounds:
- Reaction with Electrophiles: Reactions with benzeneselenenyl chloride, arenesulfenyl chlorides, and arenesulfinyl chlorides in the presence of this compound oxides can yield various 5-substituted 2,2′-bipyridyl derivatives. [, ]
- Addition to Cyanopropargylic Alcohols: In water, the addition of this compound to tertiary cyanopropargylic alcohols triggers P-C bond cleavage and oxygen insertion, resulting in the formation of (E)-cyanoallyldipyridylphosphinates. []
- Reaction with Electron-Deficient Alkynes: Reactions with electron-deficient alkynes in water yield (E)-pyridylvinylphosphine oxides, demonstrating stereoselective control in the product formation. [, ]
Q9: What are some challenges in synthesizing and handling this compound?
A9: While various synthetic routes exist, challenges include:
- Air Sensitivity: this compound is air-sensitive and can oxidize to the corresponding phosphine oxide. Handling under inert atmosphere is often required. []
- Formation of Byproducts: Traditional synthetic methods can lead to the formation of primary and secondary pyridylphosphines as byproducts, necessitating careful purification. []
Q10: How has microwave-assisted synthesis improved the preparation of this compound?
A10: Microwave irradiation has enabled a more straightforward and efficient synthesis of this compound directly from 2-bromopyridine and red phosphorus, potentially simplifying large-scale production. []
Q11: What makes certain metal complexes of this compound luminescent?
A11: The combination of this compound and its oxide with metal ions like Cu(I), Au(I), and Ag(I) can create luminescent complexes. The observed luminescence often originates from metal-to-ligand charge transfer (MLCT) and ligand-to-ligand charge transfer (LLCT) excited states within these complexes. [, , , , ]
Q12: How does the nature of the halogen atom influence luminescence in Cu(I) halide complexes of this compound?
A12: In [Cu₂(Py₃P)₂X₂] complexes (X = Cl, Br, I), the halogen atom significantly affects the balance between thermally activated delayed fluorescence (TADF) and phosphorescence. As the halogen atom changes from Cl to Br to I, the energy gap between the singlet and triplet excited states decreases, favoring TADF and suppressing phosphorescence. []
Q13: What is unique about the luminescent behavior of heterobimetallic Au(I)-Cu(I) coordination polymers incorporating this compound?
A13: In these polymers, "long-distance" electronic transitions involving the alkynyl and this compound ligands can occur over distances exceeding 1 nm. This demonstrates the potential for designing materials with tailored photophysical properties by strategically combining different metal centers and ligands. []
Disclaimer and Information on In-Vitro Research Products
Please be aware that all articles and product information presented on BenchChem are intended solely for informational purposes. The products available for purchase on BenchChem are specifically designed for in-vitro studies, which are conducted outside of living organisms. In-vitro studies, derived from the Latin term "in glass," involve experiments performed in controlled laboratory settings using cells or tissues. It is important to note that these products are not categorized as medicines or drugs, and they have not received approval from the FDA for the prevention, treatment, or cure of any medical condition, ailment, or disease. We must emphasize that any form of bodily introduction of these products into humans or animals is strictly prohibited by law. It is essential to adhere to these guidelines to ensure compliance with legal and ethical standards in research and experimentation.