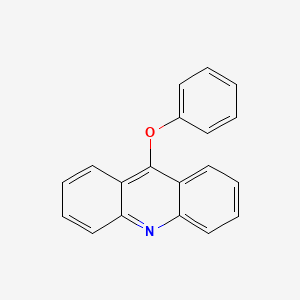
9-Phenoxyacridine
Overview
Description
9-Phenoxyacridine is an organic compound with the molecular formula C19H13NO. It belongs to the class of acridine derivatives, which are known for their wide range of applications in various fields, including medicinal chemistry, material science, and photophysics. The structure of this compound consists of an acridine core with a phenoxy group attached at the 9th position, contributing to its unique chemical properties and biological activities .
Preparation Methods
Synthetic Routes and Reaction Conditions: The synthesis of 9-Phenoxyacridine typically involves the Ullmann condensation reaction. One common method starts with the condensation of 2-chlorobenzoic acid and aniline to form 9-chloroacridine. This intermediate is then reacted with phenol in the presence of a base, such as ammonium carbonate, to yield this compound . The reaction conditions include heating the mixture to around 120°C and maintaining this temperature for a specific duration to ensure complete conversion .
Industrial Production Methods: Industrial production of this compound follows similar synthetic routes but on a larger scale. The use of continuous flow reactors and optimized reaction conditions can enhance the yield and purity of the final product. The process involves careful control of temperature, pressure, and reagent concentrations to achieve efficient production .
Chemical Reactions Analysis
Types of Reactions: 9-Phenoxyacridine undergoes various chemical reactions, including:
Oxidation: It can be oxidized to form corresponding quinone derivatives.
Reduction: Reduction reactions can convert it into dihydro derivatives.
Substitution: It can undergo nucleophilic substitution reactions, especially at the phenoxy group.
Common Reagents and Conditions:
Oxidation: Common oxidizing agents include potassium permanganate and chromium trioxide.
Reduction: Reducing agents such as sodium borohydride and lithium aluminum hydride are used.
Substitution: Reagents like sodium hydroxide and potassium carbonate are employed in substitution reactions.
Major Products: The major products formed from these reactions include various substituted acridine derivatives, which can exhibit different biological and chemical properties .
Scientific Research Applications
9-Phenoxyacridine has a wide range of applications in scientific research:
Mechanism of Action
The mechanism of action of 9-Phenoxyacridine primarily involves its ability to intercalate into double-stranded DNA. This intercalation disrupts the helical structure of DNA, affecting processes such as replication and transcription. The compound can also inhibit enzymes like topoisomerase and telomerase, which are crucial for DNA maintenance and cell proliferation . These interactions make it a potential candidate for anticancer therapies .
Comparison with Similar Compounds
9-Chloroacridine: Similar in structure but with a chlorine atom instead of a phenoxy group.
Acriflavine: An acridine derivative with antibacterial properties, used as a disinfectant.
Proflavine: Another acridine derivative known for its antiseptic properties.
Uniqueness: 9-Phenoxyacridine stands out due to its phenoxy group, which enhances its ability to interact with biological molecules and its potential as a therapeutic agent. Its unique structure allows for diverse chemical modifications, making it a versatile compound in scientific research .
Properties
IUPAC Name |
9-phenoxyacridine | |
---|---|---|
Details | Computed by Lexichem TK 2.7.0 (PubChem release 2021.05.07) | |
Source | PubChem | |
URL | https://pubchem.ncbi.nlm.nih.gov | |
Description | Data deposited in or computed by PubChem | |
InChI |
InChI=1S/C19H13NO/c1-2-8-14(9-3-1)21-19-15-10-4-6-12-17(15)20-18-13-7-5-11-16(18)19/h1-13H | |
Details | Computed by InChI 1.0.6 (PubChem release 2021.05.07) | |
Source | PubChem | |
URL | https://pubchem.ncbi.nlm.nih.gov | |
Description | Data deposited in or computed by PubChem | |
InChI Key |
MFCLDPQGJMEEBE-UHFFFAOYSA-N | |
Details | Computed by InChI 1.0.6 (PubChem release 2021.05.07) | |
Source | PubChem | |
URL | https://pubchem.ncbi.nlm.nih.gov | |
Description | Data deposited in or computed by PubChem | |
Canonical SMILES |
C1=CC=C(C=C1)OC2=C3C=CC=CC3=NC4=CC=CC=C42 | |
Details | Computed by OEChem 2.3.0 (PubChem release 2021.05.07) | |
Source | PubChem | |
URL | https://pubchem.ncbi.nlm.nih.gov | |
Description | Data deposited in or computed by PubChem | |
Molecular Formula |
C19H13NO | |
Details | Computed by PubChem 2.1 (PubChem release 2021.05.07) | |
Source | PubChem | |
URL | https://pubchem.ncbi.nlm.nih.gov | |
Description | Data deposited in or computed by PubChem | |
DSSTOX Substance ID |
DTXSID80175760 | |
Record name | Acridine, 9-phenoxy- | |
Source | EPA DSSTox | |
URL | https://comptox.epa.gov/dashboard/DTXSID80175760 | |
Description | DSSTox provides a high quality public chemistry resource for supporting improved predictive toxicology. | |
Molecular Weight |
271.3 g/mol | |
Details | Computed by PubChem 2.1 (PubChem release 2021.05.07) | |
Source | PubChem | |
URL | https://pubchem.ncbi.nlm.nih.gov | |
Description | Data deposited in or computed by PubChem | |
CAS No. |
2148-14-3 | |
Record name | Acridine, 9-phenoxy- | |
Source | ChemIDplus | |
URL | https://pubchem.ncbi.nlm.nih.gov/substance/?source=chemidplus&sourceid=0002148143 | |
Description | ChemIDplus is a free, web search system that provides access to the structure and nomenclature authority files used for the identification of chemical substances cited in National Library of Medicine (NLM) databases, including the TOXNET system. | |
Record name | Acridine, 9-phenoxy- | |
Source | DTP/NCI | |
URL | https://dtp.cancer.gov/dtpstandard/servlet/dwindex?searchtype=NSC&outputformat=html&searchlist=337974 | |
Description | The NCI Development Therapeutics Program (DTP) provides services and resources to the academic and private-sector research communities worldwide to facilitate the discovery and development of new cancer therapeutic agents. | |
Explanation | Unless otherwise indicated, all text within NCI products is free of copyright and may be reused without our permission. Credit the National Cancer Institute as the source. | |
Record name | Acridine, 9-phenoxy- | |
Source | EPA DSSTox | |
URL | https://comptox.epa.gov/dashboard/DTXSID80175760 | |
Description | DSSTox provides a high quality public chemistry resource for supporting improved predictive toxicology. | |
Record name | 9-Phenoxyacridine | |
Source | FDA Global Substance Registration System (GSRS) | |
URL | https://gsrs.ncats.nih.gov/ginas/app/beta/substances/K5Q5A5UL8M | |
Description | The FDA Global Substance Registration System (GSRS) enables the efficient and accurate exchange of information on what substances are in regulated products. Instead of relying on names, which vary across regulatory domains, countries, and regions, the GSRS knowledge base makes it possible for substances to be defined by standardized, scientific descriptions. | |
Explanation | Unless otherwise noted, the contents of the FDA website (www.fda.gov), both text and graphics, are not copyrighted. They are in the public domain and may be republished, reprinted and otherwise used freely by anyone without the need to obtain permission from FDA. Credit to the U.S. Food and Drug Administration as the source is appreciated but not required. | |
Synthesis routes and methods
Procedure details
Retrosynthesis Analysis
AI-Powered Synthesis Planning: Our tool employs the Template_relevance Pistachio, Template_relevance Bkms_metabolic, Template_relevance Pistachio_ringbreaker, Template_relevance Reaxys, Template_relevance Reaxys_biocatalysis model, leveraging a vast database of chemical reactions to predict feasible synthetic routes.
One-Step Synthesis Focus: Specifically designed for one-step synthesis, it provides concise and direct routes for your target compounds, streamlining the synthesis process.
Accurate Predictions: Utilizing the extensive PISTACHIO, BKMS_METABOLIC, PISTACHIO_RINGBREAKER, REAXYS, REAXYS_BIOCATALYSIS database, our tool offers high-accuracy predictions, reflecting the latest in chemical research and data.
Strategy Settings
Precursor scoring | Relevance Heuristic |
---|---|
Min. plausibility | 0.01 |
Model | Template_relevance |
Template Set | Pistachio/Bkms_metabolic/Pistachio_ringbreaker/Reaxys/Reaxys_biocatalysis |
Top-N result to add to graph | 6 |
Feasible Synthetic Routes
Disclaimer and Information on In-Vitro Research Products
Please be aware that all articles and product information presented on BenchChem are intended solely for informational purposes. The products available for purchase on BenchChem are specifically designed for in-vitro studies, which are conducted outside of living organisms. In-vitro studies, derived from the Latin term "in glass," involve experiments performed in controlled laboratory settings using cells or tissues. It is important to note that these products are not categorized as medicines or drugs, and they have not received approval from the FDA for the prevention, treatment, or cure of any medical condition, ailment, or disease. We must emphasize that any form of bodily introduction of these products into humans or animals is strictly prohibited by law. It is essential to adhere to these guidelines to ensure compliance with legal and ethical standards in research and experimentation.