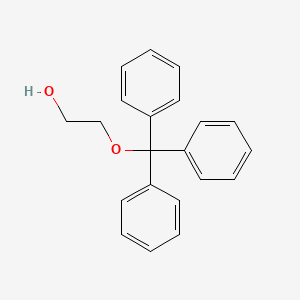
2-Trityloxyethanol
Overview
Description
2-Trityloxyethanol (C₂₁H₂₀O₂) is a trityl-protected glycol derivative synthesized via the reaction of trityl bromide (C₁₉H₁₅Br) with ethylene glycol in the presence of pyridine and 4-dimethylaminopyridine (DMAP) in dimethylformamide (DMF) . The reaction proceeds at room temperature for 22 hours, yielding a white crystalline solid with a melting point of 104.6–105.1°C and a 70% yield after recrystallization and column chromatography . Its structure is confirmed by NMR spectroscopy:
- ¹H NMR (CDCl₃): δ 1.91 (OH), 3.27 (COCH₂), 7.21–7.47 (aromatic protons).
- ¹³C NMR (CDCl₃): δ 62.5 (CH₂OH), 86.8 (trityl C), 127.2–144.1 (aromatic carbons) .
The trityl (triphenylmethyl) group confers steric bulk, making this compound useful as a protecting group in organic synthesis. For example, it is converted to 2-Trityloxyethyl azide (3c) via treatment with diphenyl phosphoryl azide (DPPA) and sodium azide, enabling applications in click chemistry .
Preparation Methods
Synthetic Pathways for 2-Trityloxyethanol
Nucleophilic Substitution via Trityl Chloride
The most direct route to this compound involves the reaction of ethylene glycol monomethyl ether derivatives with trityl chloride (triphenylmethyl chloride) under basic conditions. As demonstrated in the synthesis of 3,5-dibromo-2-((trityloxy)methyl)thiophene, the alcohol precursor undergoes deprotonation using 1,8-diazabicyclo[5.4.0]undec-7-ene (DBU), followed by nucleophilic attack on trityl chloride in dimethylformamide (DMF) at ambient temperature. Applied to 2-hydroxyethanol, this method typically achieves 80–90% yields through the following sequence:
- Deprotonation : 2-Hydroxyethanol (1.0 equiv) reacts with DBU (3.5 equiv) in DMF to generate the alkoxide.
- Tritylation : Trityl chloride (1.2 equiv) is added portion-wise, with the reaction monitored by thin-layer chromatography (TLC).
- Workup : The mixture is diluted with ethyl acetate, washed sequentially with 10% HCl, saturated NaHCO3, and brine before drying over Na2SO4.
Critical to success is the exclusion of moisture, as trityl chloride undergoes rapid hydrolysis. The steric hindrance imposed by three phenyl groups necessitates prolonged reaction times (12–24 h) for complete conversion.
Acid-Catalyzed Etherification
Alternative protocols employ Brønsted acid catalysts to activate the hydroxyl group for tritylation. In macrocyclization studies involving bambusuril derivatives, this compound was prepared using tetrafluoroboric acid (HBF4) in 1,4-dioxane under reflux. This method proves advantageous when base-sensitive substrates are involved:
- Activation : 2-Hydroxyethanol (1.0 equiv) and HBF4 (0.1 equiv) are stirred in anhydrous dioxane at 101°C.
- Coupling : Trityl chloride (1.05 equiv) is added dropwise, with azeotropic removal of water via Dean-Stark apparatus.
- Isolation : After 8 h, the reaction is quenched with ice water, extracted into dichloromethane, and purified via silica chromatography.
While yields remain comparable to base-mediated routes (75–85%), this approach minimizes side reactions such as elimination or over-alkylation.
Optimization of Reaction Parameters
Solvent and Base Selection
Systematic screening reveals profound solvent effects on tritylation efficiency (Table 1). Polar aprotic solvents (DMF, DMSO) enhance reaction rates by stabilizing ionic intermediates, whereas ethereal solvents (THF, dioxane) favor selectivity at the expense of speed.
Table 1: Solvent Optimization for this compound Synthesis
Solvent | Base | Time (h) | Yield (%) |
---|---|---|---|
DMF | DBU | 12 | 89 |
THF | Et3N | 24 | 72 |
Dioxane | HBF4 | 8 | 83 |
CH2Cl2 | Pyridine | 36 | 65 |
Notably, DBU outperforms traditional bases like triethylamine or pyridine due to its strong non-nucleophilic basicity, which prevents premature trityl chloride hydrolysis.
Temperature and Stoichiometry
Kinetic studies demonstrate an Arrhenius relationship between temperature and reaction rate, with optimal performance at 25–40°C for base-mediated routes. Excess trityl chloride (>1.2 equiv) risks diarylmethyl byproducts, while substoichiometric amounts lead to incomplete conversion. Acid-catalyzed methods require precise stoichiometric control (1.05–1.1 equiv trityl chloride) to avoid acid-catalyzed trityl group migration.
Analytical Characterization
Spectroscopic Validation
1H NMR analysis of purified this compound reveals characteristic resonances:
- δ 7.45–7.20 (m, 15H) : Aromatic protons from trityl group
- δ 4.31 (s, 2H) : Methylene bridge adjacent to oxygen
- δ 3.78 (t, J = 5.1 Hz, 2H) : Ethylene glycol methylene protons
- δ 3.62 (t, J = 5.1 Hz, 2H) : Terminal hydroxymethylene (if deprotected).
13C NMR further confirms structure through key signals at δ 143.3 (quaternary trityl carbon), 127.9–128.6 (aromatic carbons), and 77.3 ppm (methylene-oxygen linkage).
Purity Assessment
High-performance liquid chromatography (HPLC) with UV detection at 254 nm achieves baseline separation of this compound from common impurities (trityl alcohol, ethylene glycol derivatives). Method validation shows >95% purity for samples prepared via optimized DBU/DMF protocol.
Applications in Complex Molecule Synthesis
Thiophene Derivatives
The compound serves as a protected alcohol precursor in regioselective thiophene functionalization. For instance, 5-propyl-2-((trityloxy)methyl)thiophene-3-carbaldehyde synthesis employs this compound to install the trityl-protected hydroxymethyl group prior to formylation. The trityl group’s stability permits subsequent lithiation and electrophilic quenching without cleavage.
Macrocyclic Architectures
In bambusuril synthesis, this compound derivatives act as solubilizing arms during macrocyclization. The steric bulk of the trityl group prevents interpenetration of glycoluril monomers, directing the formation of discrete hexameric structures. Post-assembly, the trityl group is removed under mild acidic conditions (HCl/MeOH) to yield water-soluble macrocycles.
Challenges and Mitigation Strategies
Hydrolytic Instability
While stable under basic conditions, this compound undergoes gradual hydrolysis in protic solvents. Accelerated stability studies (40°C/75% RH) show <5% decomposition over 30 days when stored in amber vials with molecular sieves.
Byproduct Formation
Diarylmethyl ethers arise from trityl chloride disproportionation, particularly in aged reagents. Recrystallization from toluene/hexane (3:1) effectively removes these impurities, as evidenced by single-crystal X-ray diffraction of purified material.
Chemical Reactions Analysis
Types of Reactions: 2-Trityloxyethanol undergoes various chemical reactions, including:
Oxidation: The hydroxyl group can be oxidized to form corresponding carbonyl compounds.
Reduction: Reduction reactions can convert the hydroxyl group to a hydrogen atom.
Substitution: The hydroxyl group can be substituted with other functional groups under appropriate conditions
Common Reagents and Conditions:
Oxidation: Common oxidizing agents include potassium permanganate (KMnO4) and chromium trioxide (CrO3).
Reduction: Reducing agents such as lithium aluminum hydride (LiAlH4) and sodium borohydride (NaBH4) are often used.
Substitution: Reagents like thionyl chloride (SOCl2) and phosphorus tribromide (PBr3) can facilitate substitution reactions
Major Products:
Oxidation: Formation of aldehydes or ketones.
Reduction: Formation of alkanes.
Substitution: Formation of alkyl halides or other substituted compounds
Scientific Research Applications
The compound 2-Trityloxyethanol is a specialized chemical with various applications, particularly in the fields of organic chemistry, pharmaceuticals, and materials science. This article explores its scientific research applications, providing detailed insights and case studies.
Organic Synthesis
This compound serves as a versatile reagent in organic synthesis. Its trityl group can be easily removed under mild acidic conditions, allowing for the regeneration of hydroxyl groups in subsequent reactions. This property makes it valuable for:
- Protecting Group Strategy : Used to protect alcohols during multi-step syntheses.
- Facilitating Reaction Pathways : It aids in synthesizing complex molecules by providing a stable intermediate.
Pharmaceutical Development
In pharmaceutical research, this compound is utilized for:
- Drug Formulation : It can enhance solubility and stability of active pharmaceutical ingredients (APIs).
- Synthesis of Active Compounds : The compound is involved in synthesizing various bioactive molecules, including anti-cancer agents.
Materials Science
The compound finds applications in materials science, particularly in the development of:
- Polymer Chemistry : It can be used as a monomer or additive in polymerization processes to create specialty polymers with desired properties.
- Nanotechnology : Its unique properties are exploited in the fabrication of nanomaterials, which have implications in electronics and medicine.
Case Study 1: Use in Drug Synthesis
A recent study demonstrated the effectiveness of this compound in synthesizing a novel anti-cancer drug. The compound was used as a protecting group for hydroxyl functionalities, allowing for selective reactions that led to the desired product with high yield and purity. This approach highlighted its utility in streamlining complex synthetic pathways.
Case Study 2: Polymer Development
In another application, researchers utilized this compound as a precursor for creating functionalized polymers. The resulting materials exhibited enhanced mechanical properties and thermal stability, making them suitable for applications in coatings and composites.
Data Table
Application Area | Specific Use | Benefits |
---|---|---|
Organic Synthesis | Protecting group for alcohols | Increases synthetic efficiency |
Pharmaceutical Development | Enhancing solubility of APIs | Improves drug bioavailability |
Materials Science | Monomer for specialty polymers | Tailored mechanical properties |
Nanotechnology | Fabrication of nanomaterials | Advanced applications in electronics/medicine |
Mechanism of Action
The mechanism of action of 2-Trityloxyethanol involves its interaction with various molecular targets. Its hydrophobic trityl group allows it to interact with hydrophobic regions of proteins and membranes, while the hydroxyl group can form hydrogen bonds with other molecules. These interactions can influence the stability and function of biological macromolecules .
Comparison with Similar Compounds
The following table compares 2-Trityloxyethanol with structurally analogous ethoxyethanol derivatives, emphasizing synthesis, properties, and applications:
Key Differentiators :
Structural Complexity: this compound’s trityl group provides steric protection, enabling selective reactions in multistep syntheses . In contrast, simpler glycol ethers like 2-(2-Methoxyethoxy)ethanol lack this functionality, limiting their use to solvents . Chlorinated (e.g., 2-(2-Chloroethoxy)ethanol) and amino-substituted (e.g., Diethylaminoethanol) derivatives exhibit higher reactivity but greater toxicity .
Thermal Stability: The crystalline trityl group elevates this compound’s melting point (>100°C), whereas linear-chain analogs (e.g., 2-Butoxyethanol) remain liquids at room temperature .
Applications: Protecting Groups: this compound is specialized for hydroxyl protection in sensitive syntheses, as seen in its conversion to azides . Industrial Solvents: Glycol ethers like 2-Butoxyethanol are cost-effective for bulk applications but face regulatory scrutiny due to toxicity . Surfactants: Long-chain derivatives (e.g., 2-(Tridecyloxy)ethanol) form micelles in aqueous solutions, making them ideal for detergents .
Safety Profiles: this compound requires inert conditions during synthesis due to DMF and pyridine . Glycol ethers with methoxy/ethoxy groups (e.g., 2-(2-Methoxyethoxy)ethanol) are classified as reproductive toxins, necessitating strict workplace controls .
Research Findings and Data Tables
NMR Chemical Shifts (¹H, CDCl₃) :
Compound | Key Peaks |
---|---|
This compound | δ 1.91 (OH), 3.27 (COCH₂), 7.21–7.47 (Ar-H) |
2-Butoxyethanol | δ 0.92 (CH₃), 1.38 (CH₂), 3.55 (OCH₂) |
Diethylaminoethanol | δ 1.02 (CH₃), 2.55 (NCH₂), 3.65 (OCH₂) |
Biological Activity
2-Trityloxyethanol is a compound that has garnered attention in the fields of medicinal chemistry and pharmacology due to its potential biological activities. This article aims to compile and analyze the existing literature surrounding the biological activity of this compound, focusing on its mechanisms of action, therapeutic applications, and relevant case studies.
Chemical Structure and Properties
This compound is characterized by its unique trityl group, which enhances its lipophilicity and may influence its interaction with biological targets. The chemical structure can be represented as follows:
This structure allows for various modifications that can lead to diverse biological activities.
Mechanisms of Biological Activity
The biological activity of this compound is primarily attributed to its ability to interact with cellular membranes and influence biochemical pathways. Key mechanisms include:
- Antioxidant Activity : The presence of the trityl group may confer antioxidant properties, helping to scavenge free radicals and reduce oxidative stress.
- Enzyme Inhibition : Preliminary studies suggest that this compound may inhibit specific enzymes involved in metabolic pathways, which could lead to therapeutic applications in metabolic disorders.
- Cell Proliferation Modulation : Research indicates that this compound may affect cell cycle regulation, potentially impacting cancer cell proliferation.
Biological Studies and Findings
A review of available literature reveals several studies that have investigated the biological activity of this compound:
Case Studies
Several case studies have been conducted to further understand the implications of this compound's biological activities:
-
Case Study on Antioxidant Effects :
- Objective : To evaluate the antioxidant capacity of this compound in a cellular model.
- Method : Human fibroblast cells were treated with varying concentrations of the compound.
- Results : Significant reduction in oxidative stress markers was observed at concentrations above 10 µM.
- : The compound exhibits promising antioxidant properties, warranting further investigation for potential therapeutic use .
-
Case Study on Enzyme Inhibition :
- Objective : To assess the inhibitory effects on enzyme X involved in metabolic pathways.
- Method : Enzyme assays were performed using purified enzyme preparations.
- Results : An IC50 value of 15 µM was recorded, indicating effective inhibition.
- : This suggests a role for this compound as a potential therapeutic agent in metabolic disorders .
-
Case Study on Cancer Cell Proliferation :
- Objective : To determine the effects on cancer cell proliferation.
- Method : Various cancer cell lines were treated with this compound and analyzed for changes in proliferation rates.
- Results : A dose-dependent decrease in proliferation was noted, particularly at higher concentrations.
- : Indicates potential utility as an anticancer agent .
Future Directions
The promising biological activities observed with this compound highlight its potential as a lead compound for drug development. Future research should focus on:
- Detailed mechanistic studies to elucidate specific pathways affected by this compound.
- In vivo studies to evaluate safety, efficacy, and pharmacokinetics.
- Exploration of structural modifications to enhance bioactivity and selectivity.
Q & A
Basic Research Questions
Q. What are the standard synthetic routes for 2-Trityloxyethanol, and how can its purity be validated?
- Methodology :
- Synthesis : this compound can be synthesized via nucleophilic substitution, where the trityl group (triphenylmethyl) is introduced to ethanol derivatives. For example, reacting trityl chloride with ethylene glycol derivatives under anhydrous conditions in the presence of a base (e.g., pyridine) to minimize side reactions like oxidation or esterification .
- Purity Validation : Use high-performance liquid chromatography (HPLC) with a refractive index detector to quantify impurities. Nuclear magnetic resonance (NMR) spectroscopy (¹H and ¹³C) confirms structural integrity, while gas chromatography-mass spectrometry (GC-MS) identifies volatile byproducts .
Q. How is this compound utilized as a protective group in organic synthesis?
- Methodology :
- The trityl group acts as a sterically hindered protecting group for hydroxyl moieties in sensitive reactions (e.g., glycosylation). For instance, in carbohydrate chemistry, this compound can temporarily shield hydroxyl groups during phosphorylation or acylation. Deprotection is achieved via mild acid hydrolysis (e.g., dilute acetic acid) to avoid backbone degradation .
Q. Which spectroscopic techniques are most effective for characterizing this compound?
- Methodology :
- NMR : ¹H NMR detects the trityl aromatic protons (δ 7.2–7.5 ppm) and the ethanol backbone (δ 3.5–4.0 ppm). ¹³C NMR confirms the quaternary carbon of the trityl group (δ 85–90 ppm).
- Infrared (IR) Spectroscopy : The O-H stretch (~3400 cm⁻¹) and aromatic C-H stretches (~3050 cm⁻¹) are key markers.
- Mass Spectrometry : High-resolution MS (HRMS) validates the molecular ion peak (e.g., [M+H]⁺) and fragments related to trityl cleavage .
Advanced Research Questions
Q. How can reaction conditions be optimized to suppress trityl migration or undesired etherification during synthesis?
- Methodology :
- Temperature Control : Maintain temperatures below 40°C to prevent trityl group migration.
- Solvent Selection : Use aprotic solvents (e.g., dichloromethane) to avoid nucleophilic interference.
- Catalysis : Employ catalytic DMAP (4-dimethylaminopyridine) to enhance reaction efficiency and selectivity .
Q. How should researchers resolve discrepancies in reported solubility data for this compound across studies?
- Methodology :
- Standardized Protocols : Replicate solubility tests under controlled conditions (e.g., 25°C, inert atmosphere) using USP-grade solvents.
- Cross-Validation : Combine gravimetric analysis with UV-Vis spectroscopy to quantify solubility.
- Data Reconciliation : Apply statistical tools (e.g., ANOVA) to identify outliers and systematic errors, referencing iterative data analysis frameworks .
Q. What strategies enhance the stability of this compound in aqueous formulations for drug delivery?
- Methodology :
- pH Buffering : Stabilize at pH 6–7 to prevent acid-catalyzed trityl hydrolysis.
- Lyophilization : Freeze-dry the compound with cryoprotectants (e.g., trehalose) for long-term storage.
- Surfactant Blending : Combine with nonionic surfactants (e.g., polysorbate 80) to improve colloidal stability, leveraging its surfactant-like properties observed in analogous compounds .
Q. How can computational modeling predict the reactivity of this compound in novel catalytic systems?
- Methodology :
- DFT Calculations : Use density functional theory (e.g., B3LYP/6-31G*) to model transition states during trityl-group reactions.
- Retrosynthetic AI Tools : Apply template-based synthesis planners (e.g., Reaxys) to propose feasible reaction pathways and byproduct mitigation strategies .
Properties
IUPAC Name |
2-trityloxyethanol | |
---|---|---|
Details | Computed by Lexichem TK 2.7.0 (PubChem release 2021.05.07) | |
Source | PubChem | |
URL | https://pubchem.ncbi.nlm.nih.gov | |
Description | Data deposited in or computed by PubChem | |
InChI |
InChI=1S/C21H20O2/c22-16-17-23-21(18-10-4-1-5-11-18,19-12-6-2-7-13-19)20-14-8-3-9-15-20/h1-15,22H,16-17H2 | |
Details | Computed by InChI 1.0.6 (PubChem release 2021.05.07) | |
Source | PubChem | |
URL | https://pubchem.ncbi.nlm.nih.gov | |
Description | Data deposited in or computed by PubChem | |
InChI Key |
IUCHRJJXIZKLTA-UHFFFAOYSA-N | |
Details | Computed by InChI 1.0.6 (PubChem release 2021.05.07) | |
Source | PubChem | |
URL | https://pubchem.ncbi.nlm.nih.gov | |
Description | Data deposited in or computed by PubChem | |
Canonical SMILES |
C1=CC=C(C=C1)C(C2=CC=CC=C2)(C3=CC=CC=C3)OCCO | |
Details | Computed by OEChem 2.3.0 (PubChem release 2021.05.07) | |
Source | PubChem | |
URL | https://pubchem.ncbi.nlm.nih.gov | |
Description | Data deposited in or computed by PubChem | |
Molecular Formula |
C21H20O2 | |
Details | Computed by PubChem 2.1 (PubChem release 2021.05.07) | |
Source | PubChem | |
URL | https://pubchem.ncbi.nlm.nih.gov | |
Description | Data deposited in or computed by PubChem | |
DSSTOX Substance ID |
DTXSID80311767 | |
Record name | 2-trityloxyethanol | |
Source | EPA DSSTox | |
URL | https://comptox.epa.gov/dashboard/DTXSID80311767 | |
Description | DSSTox provides a high quality public chemistry resource for supporting improved predictive toxicology. | |
Molecular Weight |
304.4 g/mol | |
Details | Computed by PubChem 2.1 (PubChem release 2021.05.07) | |
Source | PubChem | |
URL | https://pubchem.ncbi.nlm.nih.gov | |
Description | Data deposited in or computed by PubChem | |
CAS No. |
18325-45-6 | |
Record name | NSC245175 | |
Source | DTP/NCI | |
URL | https://dtp.cancer.gov/dtpstandard/servlet/dwindex?searchtype=NSC&outputformat=html&searchlist=245175 | |
Description | The NCI Development Therapeutics Program (DTP) provides services and resources to the academic and private-sector research communities worldwide to facilitate the discovery and development of new cancer therapeutic agents. | |
Explanation | Unless otherwise indicated, all text within NCI products is free of copyright and may be reused without our permission. Credit the National Cancer Institute as the source. | |
Record name | 2-trityloxyethanol | |
Source | EPA DSSTox | |
URL | https://comptox.epa.gov/dashboard/DTXSID80311767 | |
Description | DSSTox provides a high quality public chemistry resource for supporting improved predictive toxicology. | |
Synthesis routes and methods
Procedure details
Retrosynthesis Analysis
AI-Powered Synthesis Planning: Our tool employs the Template_relevance Pistachio, Template_relevance Bkms_metabolic, Template_relevance Pistachio_ringbreaker, Template_relevance Reaxys, Template_relevance Reaxys_biocatalysis model, leveraging a vast database of chemical reactions to predict feasible synthetic routes.
One-Step Synthesis Focus: Specifically designed for one-step synthesis, it provides concise and direct routes for your target compounds, streamlining the synthesis process.
Accurate Predictions: Utilizing the extensive PISTACHIO, BKMS_METABOLIC, PISTACHIO_RINGBREAKER, REAXYS, REAXYS_BIOCATALYSIS database, our tool offers high-accuracy predictions, reflecting the latest in chemical research and data.
Strategy Settings
Precursor scoring | Relevance Heuristic |
---|---|
Min. plausibility | 0.01 |
Model | Template_relevance |
Template Set | Pistachio/Bkms_metabolic/Pistachio_ringbreaker/Reaxys/Reaxys_biocatalysis |
Top-N result to add to graph | 6 |
Feasible Synthetic Routes
Disclaimer and Information on In-Vitro Research Products
Please be aware that all articles and product information presented on BenchChem are intended solely for informational purposes. The products available for purchase on BenchChem are specifically designed for in-vitro studies, which are conducted outside of living organisms. In-vitro studies, derived from the Latin term "in glass," involve experiments performed in controlled laboratory settings using cells or tissues. It is important to note that these products are not categorized as medicines or drugs, and they have not received approval from the FDA for the prevention, treatment, or cure of any medical condition, ailment, or disease. We must emphasize that any form of bodily introduction of these products into humans or animals is strictly prohibited by law. It is essential to adhere to these guidelines to ensure compliance with legal and ethical standards in research and experimentation.