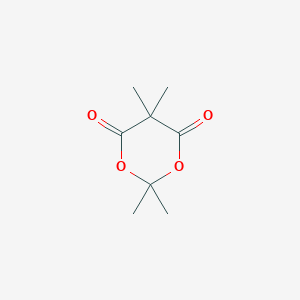
2,2,5,5-Tetramethyl-1,3-dioxane-4,6-dione
Overview
Description
2,2,5,5-Tetramethyl-1,3-dioxane-4,6-dione is an organic compound with the molecular formula C8H12O4. It is a heterocyclic compound featuring a dioxane ring substituted with four methyl groups. This compound is known for its stability and unique reactivity, making it a valuable intermediate in various chemical syntheses .
Mechanism of Action
Mode of Action
It has been used for trapping the adduct formed during the reaction between alkyl isocyanides and dialkyl acetylenedicarboxylates . This suggests that it may interact with its targets through covalent bonding, leading to changes in the targets’ structure and function.
Biochemical Pathways
Its use in trapping adducts suggests that it may be involved in reactions related to alkyl isocyanides and dialkyl acetylenedicarboxylates . The downstream effects of these interactions would depend on the specific roles of these compounds in cellular processes.
Result of Action
Its role in trapping adducts suggests that it may alter the structure and function of its targets, potentially leading to changes in cellular processes .
Preparation Methods
Synthetic Routes and Reaction Conditions
2,2,5,5-Tetramethyl-1,3-dioxane-4,6-dione can be synthesized through the reaction of acetone with malonic acid in the presence of a strong acid catalyst. The reaction typically involves heating the mixture under reflux conditions to facilitate the formation of the dioxane ring .
Industrial Production Methods
In industrial settings, the production of this compound often involves continuous flow processes to ensure high yield and purity. The use of automated reactors and precise control of reaction parameters such as temperature and pH are crucial for efficient production .
Chemical Reactions Analysis
Types of Reactions
2,2,5,5-Tetramethyl-1,3-dioxane-4,6-dione undergoes various chemical reactions, including:
Oxidation: The compound can be oxidized to form corresponding carboxylic acids.
Reduction: Reduction reactions can convert it into alcohols or other reduced forms.
Substitution: It can undergo nucleophilic substitution reactions, where one of the methyl groups is replaced by another functional group.
Common Reagents and Conditions
Oxidation: Common oxidizing agents include potassium permanganate (KMnO4) and chromium trioxide (CrO3).
Reduction: Reducing agents such as lithium aluminum hydride (LiAlH4) and sodium borohydride (NaBH4) are typically used.
Substitution: Nucleophiles like amines or thiols can be used under basic conditions to facilitate substitution reactions.
Major Products
The major products formed from these reactions depend on the specific reagents and conditions used. For example, oxidation typically yields carboxylic acids, while reduction can produce alcohols .
Scientific Research Applications
2,2,5,5-Tetramethyl-1,3-dioxane-4,6-dione has a wide range of applications in scientific research:
Chemistry: It is used as an intermediate in the synthesis of complex organic molecules and as a reagent in various organic reactions.
Biology: The compound is employed in the study of enzyme mechanisms and as a probe for investigating biological pathways.
Industry: The compound is used in the production of polymers, resins, and other industrial chemicals.
Comparison with Similar Compounds
Similar Compounds
2,2,5-Trimethyl-1,3-dioxane-4,6-dione: This compound is similar in structure but has one less methyl group, which affects its reactivity and applications.
Methyl Meldrum’s acid: Another related compound, known for its use in organic synthesis and as a reagent in various chemical reactions.
Uniqueness
2,2,5,5-Tetramethyl-1,3-dioxane-4,6-dione is unique due to its high stability and specific reactivity profile, which makes it particularly useful in the synthesis of complex organic molecules and in various industrial applications .
Properties
IUPAC Name |
2,2,5,5-tetramethyl-1,3-dioxane-4,6-dione | |
---|---|---|
Details | Computed by LexiChem 2.6.6 (PubChem release 2019.06.18) | |
Source | PubChem | |
URL | https://pubchem.ncbi.nlm.nih.gov | |
Description | Data deposited in or computed by PubChem | |
InChI |
InChI=1S/C8H12O4/c1-7(2)5(9)11-8(3,4)12-6(7)10/h1-4H3 | |
Details | Computed by InChI 1.0.5 (PubChem release 2019.06.18) | |
Source | PubChem | |
URL | https://pubchem.ncbi.nlm.nih.gov | |
Description | Data deposited in or computed by PubChem | |
InChI Key |
YIAOLYVBBMEBIJ-UHFFFAOYSA-N | |
Details | Computed by InChI 1.0.5 (PubChem release 2019.06.18) | |
Source | PubChem | |
URL | https://pubchem.ncbi.nlm.nih.gov | |
Description | Data deposited in or computed by PubChem | |
Canonical SMILES |
CC1(C(=O)OC(OC1=O)(C)C)C | |
Details | Computed by OEChem 2.1.5 (PubChem release 2019.06.18) | |
Source | PubChem | |
URL | https://pubchem.ncbi.nlm.nih.gov | |
Description | Data deposited in or computed by PubChem | |
Molecular Formula |
C8H12O4 | |
Details | Computed by PubChem 2.1 (PubChem release 2019.06.18) | |
Source | PubChem | |
URL | https://pubchem.ncbi.nlm.nih.gov | |
Description | Data deposited in or computed by PubChem | |
DSSTOX Substance ID |
DTXSID00327567 | |
Record name | NSC666539 | |
Source | EPA DSSTox | |
URL | https://comptox.epa.gov/dashboard/DTXSID00327567 | |
Description | DSSTox provides a high quality public chemistry resource for supporting improved predictive toxicology. | |
Molecular Weight |
172.18 g/mol | |
Details | Computed by PubChem 2.1 (PubChem release 2021.05.07) | |
Source | PubChem | |
URL | https://pubchem.ncbi.nlm.nih.gov | |
Description | Data deposited in or computed by PubChem | |
CAS No. |
15568-97-5 | |
Record name | NSC666539 | |
Source | EPA DSSTox | |
URL | https://comptox.epa.gov/dashboard/DTXSID00327567 | |
Description | DSSTox provides a high quality public chemistry resource for supporting improved predictive toxicology. | |
Synthesis routes and methods
Procedure details
Retrosynthesis Analysis
AI-Powered Synthesis Planning: Our tool employs the Template_relevance Pistachio, Template_relevance Bkms_metabolic, Template_relevance Pistachio_ringbreaker, Template_relevance Reaxys, Template_relevance Reaxys_biocatalysis model, leveraging a vast database of chemical reactions to predict feasible synthetic routes.
One-Step Synthesis Focus: Specifically designed for one-step synthesis, it provides concise and direct routes for your target compounds, streamlining the synthesis process.
Accurate Predictions: Utilizing the extensive PISTACHIO, BKMS_METABOLIC, PISTACHIO_RINGBREAKER, REAXYS, REAXYS_BIOCATALYSIS database, our tool offers high-accuracy predictions, reflecting the latest in chemical research and data.
Strategy Settings
Precursor scoring | Relevance Heuristic |
---|---|
Min. plausibility | 0.01 |
Model | Template_relevance |
Template Set | Pistachio/Bkms_metabolic/Pistachio_ringbreaker/Reaxys/Reaxys_biocatalysis |
Top-N result to add to graph | 6 |
Feasible Synthetic Routes
Disclaimer and Information on In-Vitro Research Products
Please be aware that all articles and product information presented on BenchChem are intended solely for informational purposes. The products available for purchase on BenchChem are specifically designed for in-vitro studies, which are conducted outside of living organisms. In-vitro studies, derived from the Latin term "in glass," involve experiments performed in controlled laboratory settings using cells or tissues. It is important to note that these products are not categorized as medicines or drugs, and they have not received approval from the FDA for the prevention, treatment, or cure of any medical condition, ailment, or disease. We must emphasize that any form of bodily introduction of these products into humans or animals is strictly prohibited by law. It is essential to adhere to these guidelines to ensure compliance with legal and ethical standards in research and experimentation.