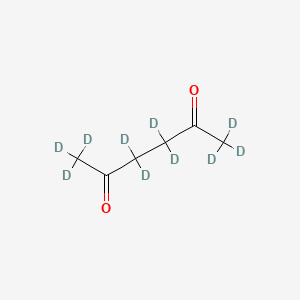
2,5-Hexanedione-D10
Overview
Description
2,5-Hexanedione-D10 is a deuterated form of 2,5-Hexanedione, a compound with the molecular formula C₆D₁₀O₂. This compound is an aliphatic diketone and is often used in scientific research due to its unique properties. The deuterium labeling (D10) makes it particularly useful in various analytical and mechanistic studies.
Mechanism of Action
Target of Action
2,5-Hexanedione-D10 is the deuterium labeled 2,5-Hexanedione . It’s primarily targeted at nerve tissues, where it induces apoptosis .
Mode of Action
The compound interacts with its targets by downregulating nerve growth factor (NGF) expression . This interaction leads to the inhibition of the phosphorylation of Akt and Bad, two key players in the PI3K/Akt pathway downstream of NGF .
Biochemical Pathways
The affected pathway is the PI3K/Akt signaling pathway . Downregulation of NGF expression by this compound leads to the repression of this pathway . This repression results in increased dimerization of Bad with Bcl-xL in the mitochondrial fraction, followed by the release of cytochrome c and activation of caspase-3 in the spinal cord of rats .
Pharmacokinetics
Stable heavy isotopes of hydrogen, carbon, and other elements have been incorporated into drug molecules, largely as tracers for quantitation during the drug development process . Deuteration has gained attention because of its potential to affect the pharmacokinetic and metabolic profiles of drugs .
Result of Action
The molecular effect of this compound’s action is the downregulation of NGF expression . On a cellular level, this leads to apoptosis in nerve tissues .
Biochemical Analysis
Biochemical Properties
2,5-Hexanedione-D10, like its non-deuterated counterpart, is known to interact with various biomolecules. It reacts with lysine residues in axonal proteins through Schiff base formation, followed by cyclization to form pyrroles . This interaction leads to the cross-linking and denaturation of proteins, which can disrupt axonal transport and function .
Cellular Effects
This compound has been linked to neurotoxicity, particularly in the peripheral nervous system . Studies have shown that it can induce apoptosis in neuronal cells . It has also been associated with cognitive impairment, altering the expression of various genes, miRNAs, and signaling pathways, leading to neuroinflammation, oxidative stress, and apoptosis .
Molecular Mechanism
The molecular mechanism of this compound involves its interaction with proteins at the molecular level. It reacts with lysine residues in proteins to form pyrroles . The oxidation of these pyrrole residues then leads to protein cross-linking and denaturation, disrupting the normal function of the proteins and leading to cellular damage .
Temporal Effects in Laboratory Settings
The effects of this compound can change over time in laboratory settings. For instance, it has been used as a biomarker for biological monitoring of workers exposed to n-hexane . The method involves determining the hexane metabolites in urine using capillary gas chromatography .
Dosage Effects in Animal Models
The effects of this compound can vary with different dosages in animal models. Studies have shown that it can induce cognitive impairment in leptin-knockout mice . The effects were observed to be dose-dependent, with alterations in gene expression, miRNAs, transcription factors, and signaling pathways .
Metabolic Pathways
This compound is involved in the metabolic pathways of n-hexane. It is the main active metabolite of n-hexane and mediates the neurotoxicity of the parent compound . It is formed through the metabolism of n-hexane in the body .
Preparation Methods
Synthetic Routes and Reaction Conditions: 2,5-Hexanedione-D10 can be synthesized through the deuteration of 2,5-Hexanedione. One common method involves the exchange of hydrogen atoms with deuterium in the presence of a deuterium source such as deuterium oxide (D₂O) under specific reaction conditions. The process typically requires a catalyst to facilitate the exchange reaction.
Industrial Production Methods: Industrial production of this compound involves large-scale deuteration processes. These processes are optimized to ensure high yield and purity of the deuterated compound. The reaction conditions, such as temperature, pressure, and catalyst choice, are carefully controlled to achieve the desired product.
Chemical Reactions Analysis
Types of Reactions: 2,5-Hexanedione-D10 undergoes various chemical reactions, including:
Oxidation: It can be oxidized to form carboxylic acids.
Reduction: Reduction reactions can convert it into alcohols.
Substitution: It can participate in nucleophilic substitution reactions.
Common Reagents and Conditions:
Oxidation: Common oxidizing agents include potassium permanganate (KMnO₄) and chromium trioxide (CrO₃).
Reduction: Reducing agents such as sodium borohydride (NaBH₄) and lithium aluminum hydride (LiAlH₄) are often used.
Substitution: Nucleophiles like amines and thiols can react with this compound under basic or acidic conditions.
Major Products:
Oxidation: Carboxylic acids.
Reduction: Alcohols.
Substitution: Various substituted derivatives depending on the nucleophile used.
Scientific Research Applications
2,5-Hexanedione-D10 is widely used in scientific research due to its unique properties:
Chemistry: It is used as a reagent in the synthesis of various organic compounds, including heterocycles and pyrroles.
Biology: It serves as a model compound in studies of metabolic pathways and enzyme mechanisms.
Medicine: It is used in the study of neurotoxicity and the effects of deuterium labeling on biological systems.
Industry: It is employed in the production of specialty chemicals and as a reference standard in analytical chemistry.
Comparison with Similar Compounds
2,5-Hexanedione: The non-deuterated form, which shares similar chemical properties but lacks the deuterium labeling.
2,4-Hexanedione: A structural isomer with different reactivity and applications.
2,5-Heptanedione: Another γ-diketone with distinct neurotoxic properties.
Uniqueness: 2,5-Hexanedione-D10 is unique due to its deuterium labeling, which makes it particularly useful in mechanistic studies and as a reference standard in analytical applications. The presence of deuterium atoms can influence reaction kinetics and provide insights into reaction mechanisms that are not possible with the non-deuterated form.
Properties
IUPAC Name |
1,1,1,3,3,4,4,6,6,6-decadeuteriohexane-2,5-dione | |
---|---|---|
Details | Computed by Lexichem TK 2.7.0 (PubChem release 2021.05.07) | |
Source | PubChem | |
URL | https://pubchem.ncbi.nlm.nih.gov | |
Description | Data deposited in or computed by PubChem | |
InChI |
InChI=1S/C6H10O2/c1-5(7)3-4-6(2)8/h3-4H2,1-2H3/i1D3,2D3,3D2,4D2 | |
Details | Computed by InChI 1.0.6 (PubChem release 2021.05.07) | |
Source | PubChem | |
URL | https://pubchem.ncbi.nlm.nih.gov | |
Description | Data deposited in or computed by PubChem | |
InChI Key |
OJVAMHKKJGICOG-MWUKXHIBSA-N | |
Details | Computed by InChI 1.0.6 (PubChem release 2021.05.07) | |
Source | PubChem | |
URL | https://pubchem.ncbi.nlm.nih.gov | |
Description | Data deposited in or computed by PubChem | |
Canonical SMILES |
CC(=O)CCC(=O)C | |
Details | Computed by OEChem 2.3.0 (PubChem release 2021.05.07) | |
Source | PubChem | |
URL | https://pubchem.ncbi.nlm.nih.gov | |
Description | Data deposited in or computed by PubChem | |
Isomeric SMILES |
[2H]C([2H])([2H])C(=O)C([2H])([2H])C([2H])([2H])C(=O)C([2H])([2H])[2H] | |
Details | Computed by OEChem 2.3.0 (PubChem release 2021.05.07) | |
Source | PubChem | |
URL | https://pubchem.ncbi.nlm.nih.gov | |
Description | Data deposited in or computed by PubChem | |
Molecular Formula |
C6H10O2 | |
Details | Computed by PubChem 2.1 (PubChem release 2021.05.07) | |
Source | PubChem | |
URL | https://pubchem.ncbi.nlm.nih.gov | |
Description | Data deposited in or computed by PubChem | |
Molecular Weight |
124.20 g/mol | |
Details | Computed by PubChem 2.1 (PubChem release 2021.05.07) | |
Source | PubChem | |
URL | https://pubchem.ncbi.nlm.nih.gov | |
Description | Data deposited in or computed by PubChem | |
Retrosynthesis Analysis
AI-Powered Synthesis Planning: Our tool employs the Template_relevance Pistachio, Template_relevance Bkms_metabolic, Template_relevance Pistachio_ringbreaker, Template_relevance Reaxys, Template_relevance Reaxys_biocatalysis model, leveraging a vast database of chemical reactions to predict feasible synthetic routes.
One-Step Synthesis Focus: Specifically designed for one-step synthesis, it provides concise and direct routes for your target compounds, streamlining the synthesis process.
Accurate Predictions: Utilizing the extensive PISTACHIO, BKMS_METABOLIC, PISTACHIO_RINGBREAKER, REAXYS, REAXYS_BIOCATALYSIS database, our tool offers high-accuracy predictions, reflecting the latest in chemical research and data.
Strategy Settings
Precursor scoring | Relevance Heuristic |
---|---|
Min. plausibility | 0.01 |
Model | Template_relevance |
Template Set | Pistachio/Bkms_metabolic/Pistachio_ringbreaker/Reaxys/Reaxys_biocatalysis |
Top-N result to add to graph | 6 |
Feasible Synthetic Routes
Disclaimer and Information on In-Vitro Research Products
Please be aware that all articles and product information presented on BenchChem are intended solely for informational purposes. The products available for purchase on BenchChem are specifically designed for in-vitro studies, which are conducted outside of living organisms. In-vitro studies, derived from the Latin term "in glass," involve experiments performed in controlled laboratory settings using cells or tissues. It is important to note that these products are not categorized as medicines or drugs, and they have not received approval from the FDA for the prevention, treatment, or cure of any medical condition, ailment, or disease. We must emphasize that any form of bodily introduction of these products into humans or animals is strictly prohibited by law. It is essential to adhere to these guidelines to ensure compliance with legal and ethical standards in research and experimentation.