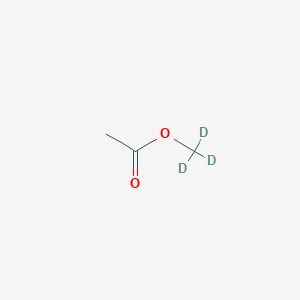
Methyl-d3 acetate
Overview
Description
Methyl-d3 acetate, also known as deuterated methyl acetate, is a chemical compound with the molecular formula C3H3D3O2. It is an isotopologue of methyl acetate, where the hydrogen atoms in the methyl group are replaced by deuterium atoms. This compound is often used in scientific research due to its unique properties, particularly in studies involving nuclear magnetic resonance (NMR) spectroscopy.
Preparation Methods
Synthetic Routes and Reaction Conditions
Methyl-d3 acetate can be synthesized through the esterification of deuterated methanol (CD3OH) with acetic acid (CH3COOH). The reaction is typically catalyzed by a strong acid, such as sulfuric acid (H2SO4), and involves heating the reactants under reflux conditions. The general reaction is as follows:
CD3OH+CH3COOH→CD3COOCH3+H2O
Industrial Production Methods
In industrial settings, the production of this compound can be optimized using microwave-assisted esterification. This method involves the use of microwave radiation to accelerate the reaction between deuterated methanol and acetic acid. Parameters such as microwave power, catalyst concentration, and reaction time are carefully controlled to achieve high yields of the desired product .
Chemical Reactions Analysis
Types of Reactions
Methyl-d3 acetate undergoes various chemical reactions, including:
Hydrolysis: In the presence of water and an acid or base catalyst, this compound can be hydrolyzed to produce deuterated methanol and acetic acid.
Transesterification: This reaction involves the exchange of the ester group with another alcohol, producing a different ester and alcohol.
Oxidation: this compound can be oxidized to produce deuterated acetic acid and other oxidation products.
Common Reagents and Conditions
Hydrolysis: Acidic or basic conditions, typically using hydrochloric acid (HCl) or sodium hydroxide (NaOH).
Transesterification: Catalysts such as sodium methoxide (NaOCH3) or sulfuric acid (H2SO4).
Oxidation: Oxidizing agents such as potassium permanganate (KMnO4) or chromium trioxide (CrO3).
Major Products Formed
Hydrolysis: Deuterated methanol (CD3OH) and acetic acid (CH3COOH).
Transesterification: Various esters and alcohols depending on the reactants used.
Oxidation: Deuterated acetic acid (CD3COOH) and other oxidation products.
Scientific Research Applications
Methyl-d3 acetate is widely used in scientific research, particularly in the following fields:
Chemistry: It is used as a solvent and reagent in various chemical reactions and studies.
Biology: this compound is used in metabolic studies to trace the incorporation of deuterium into biological molecules.
Medicine: It is used in pharmacokinetic studies to understand the metabolism and distribution of drugs.
Industry: this compound is used in the production of deuterated compounds for NMR spectroscopy and other analytical techniques.
Mechanism of Action
The mechanism of action of methyl-d3 acetate involves its interaction with various molecular targets and pathways. In NMR spectroscopy, the deuterium atoms in this compound provide distinct signals that can be used to study the structure and dynamics of molecules. The presence of deuterium also affects the chemical reactivity and physical properties of the compound, making it useful in various research applications.
Comparison with Similar Compounds
Methyl-d3 acetate can be compared with other deuterated esters, such as:
Ethyl-d5 acetate: Similar to this compound but with an ethyl group instead of a methyl group.
Propyl-d7 acetate: Contains a propyl group and seven deuterium atoms.
Butyl-d9 acetate: Contains a butyl group and nine deuterium atoms.
Uniqueness
This compound is unique due to its specific isotopic composition, which makes it particularly useful in NMR spectroscopy and other analytical techniques. Its relatively simple structure and high degree of deuteration provide clear and distinct signals in spectroscopic studies, making it a valuable tool in scientific research.
Properties
IUPAC Name |
trideuteriomethyl acetate | |
---|---|---|
Details | Computed by LexiChem 2.6.6 (PubChem release 2019.06.18) | |
Source | PubChem | |
URL | https://pubchem.ncbi.nlm.nih.gov | |
Description | Data deposited in or computed by PubChem | |
InChI |
InChI=1S/C3H6O2/c1-3(4)5-2/h1-2H3/i2D3 | |
Details | Computed by InChI 1.0.5 (PubChem release 2019.06.18) | |
Source | PubChem | |
URL | https://pubchem.ncbi.nlm.nih.gov | |
Description | Data deposited in or computed by PubChem | |
InChI Key |
KXKVLQRXCPHEJC-BMSJAHLVSA-N | |
Details | Computed by InChI 1.0.5 (PubChem release 2019.06.18) | |
Source | PubChem | |
URL | https://pubchem.ncbi.nlm.nih.gov | |
Description | Data deposited in or computed by PubChem | |
Canonical SMILES |
CC(=O)OC | |
Details | Computed by OEChem 2.1.5 (PubChem release 2019.06.18) | |
Source | PubChem | |
URL | https://pubchem.ncbi.nlm.nih.gov | |
Description | Data deposited in or computed by PubChem | |
Isomeric SMILES |
[2H]C([2H])([2H])OC(=O)C | |
Details | Computed by OEChem 2.1.5 (PubChem release 2019.06.18) | |
Source | PubChem | |
URL | https://pubchem.ncbi.nlm.nih.gov | |
Description | Data deposited in or computed by PubChem | |
Molecular Formula |
C3H6O2 | |
Details | Computed by PubChem 2.1 (PubChem release 2019.06.18) | |
Source | PubChem | |
URL | https://pubchem.ncbi.nlm.nih.gov | |
Description | Data deposited in or computed by PubChem | |
Molecular Weight |
77.10 g/mol | |
Details | Computed by PubChem 2.1 (PubChem release 2021.05.07) | |
Source | PubChem | |
URL | https://pubchem.ncbi.nlm.nih.gov | |
Description | Data deposited in or computed by PubChem | |
Retrosynthesis Analysis
AI-Powered Synthesis Planning: Our tool employs the Template_relevance Pistachio, Template_relevance Bkms_metabolic, Template_relevance Pistachio_ringbreaker, Template_relevance Reaxys, Template_relevance Reaxys_biocatalysis model, leveraging a vast database of chemical reactions to predict feasible synthetic routes.
One-Step Synthesis Focus: Specifically designed for one-step synthesis, it provides concise and direct routes for your target compounds, streamlining the synthesis process.
Accurate Predictions: Utilizing the extensive PISTACHIO, BKMS_METABOLIC, PISTACHIO_RINGBREAKER, REAXYS, REAXYS_BIOCATALYSIS database, our tool offers high-accuracy predictions, reflecting the latest in chemical research and data.
Strategy Settings
Precursor scoring | Relevance Heuristic |
---|---|
Min. plausibility | 0.01 |
Model | Template_relevance |
Template Set | Pistachio/Bkms_metabolic/Pistachio_ringbreaker/Reaxys/Reaxys_biocatalysis |
Top-N result to add to graph | 6 |
Feasible Synthetic Routes
Q1: How is Methyl-d3 acetate used to study reaction mechanisms?
A: this compound is a valuable tool for deciphering reaction pathways due to the isotopic substitution of deuterium for hydrogen in the methoxy group. By analyzing the isotopic distribution of products formed in reactions involving this compound, researchers can gain insights into the specific bond breaking and forming events that occur. For example, in the photolysis of this compound, the relative yields of CH3D and CD2O provide information about the competition between hydrogen abstraction from the acetyl and methoxy groups of this compound by methoxy radicals. [, ]
Q2: What are some examples of reactions where this compound has been used to elucidate mechanistic details?
A: this compound has proven particularly useful in studies of alkoxy radical reactions. For instance, its photolysis has been investigated at various temperatures and light intensities, providing insights into the reactions of methoxy radicals (CD3O•) with species like methane and this compound itself. [, ] Additionally, the reactions of methyl (•CH3) and trifluoromethyl (•CF3) radicals with this compound and other deuterated forms of Methyl acetate have been studied. By comparing the product distributions from these reactions, researchers have been able to determine the relative reactivities of the acetyl and methoxy groups towards these radicals. []
Q3: Has this compound been used in studies involving state-selective dissociation?
A: Yes, this compound has been utilized in research exploring site- and state-selective dissociation induced by core level excitation. Specifically, researchers have investigated its behavior in the oxygen K-edge region using time-of-flight fragment-mass spectroscopy. By analyzing the product branching ratios of ions like CH3CO+ and CDO+ from the deuterium-labeled this compound, they were able to determine that site-selective bond scission occurs at specific oxygen sites following core excitation and subsequent Auger transitions. []
Q4: What spectroscopic data is available for this compound?
A4: While the provided abstracts don't delve into specific spectroscopic details for this compound, it's important to note that techniques like NMR (Nuclear Magnetic Resonance) spectroscopy and mass spectrometry are commonly employed to characterize this compound. These techniques provide information about its structure, isotopic purity, and fragmentation patterns.
Q5: Are there any known synthetic routes to this compound?
A: One of the research papers outlines a straightforward synthesis of this compound. Starting from readily available Methyl-d3 bromide, treatment with calcium iodide yields Methyl-d3 iodide in excellent yield (94%). This deuterated methyl iodide can then be reacted with silver acetate or silver acetate-d3 to produce this compound or the fully deuterated this compound-d3, respectively. []
Disclaimer and Information on In-Vitro Research Products
Please be aware that all articles and product information presented on BenchChem are intended solely for informational purposes. The products available for purchase on BenchChem are specifically designed for in-vitro studies, which are conducted outside of living organisms. In-vitro studies, derived from the Latin term "in glass," involve experiments performed in controlled laboratory settings using cells or tissues. It is important to note that these products are not categorized as medicines or drugs, and they have not received approval from the FDA for the prevention, treatment, or cure of any medical condition, ailment, or disease. We must emphasize that any form of bodily introduction of these products into humans or animals is strictly prohibited by law. It is essential to adhere to these guidelines to ensure compliance with legal and ethical standards in research and experimentation.