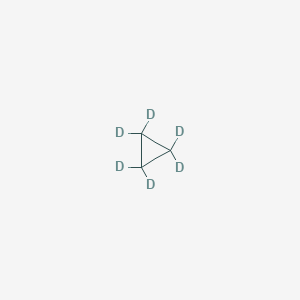
Cyclopropane-D6
Overview
Description
Cyclopropane-D6 is a deuterated form of cyclopropane, where all hydrogen atoms are replaced with deuterium. This isotopologue of cyclopropane is represented by the chemical formula C3D6. Deuterium, a stable isotope of hydrogen, has one proton and one neutron, making it twice as heavy as hydrogen. This compound is used in various scientific research applications due to its unique properties, such as increased mass and altered vibrational spectra compared to its non-deuterated counterpart .
Mechanism of Action
Target of Action
Cyclopropane-D6, like other cyclopropane derivatives, is known to interact with various targets within the body. Cyclopropane derivatives are often incorporated into drug candidates to improve potency, metabolic stability, or pharmacokinetic properties .
Mode of Action
The mode of action of this compound involves its interaction with its targets, leading to various changes. The cyclopropane fragment imposes conformational rigidity on the molecules of physiologically active compounds . On the other hand, the replacement of acyclic terminal and “linker” groups with a cyclopropane fragment increases the metabolic stability of the target structures .
Biochemical Pathways
Cyclopropane derivatives, including this compound, can be involved in various biochemical pathways. They are often incorporated into drug candidates and are usually essential for biological activities . The enzymatic cyclopropanations can be grouped into two major pathways according to whether the reaction involves an exogenous C1 unit from S-adenosylmethionine (SAM) or not .
Pharmacokinetics
The pharmacokinetic properties of this compound, such as absorption, distribution, metabolism, and excretion (ADME), are influenced by its physicochemical parameters . Cyclopropane derivatives are known to improve physicochemical properties compared to their parent aromatics, including improved solubility and reduced susceptibility to metabolism .
Result of Action
The molecular and cellular effects of this compound’s action are likely to be diverse, given the wide range of reactivities shown by cyclopropane derivatives .
Action Environment
The action, efficacy, and stability of this compound can be influenced by various environmental factors. These factors could include temperature, pH, and the presence of other molecules, which can affect the reactivity and stability of cyclopropane derivatives . .
Biochemical Analysis
Biochemical Properties
Cyclopropane-D6 plays a significant role in biochemical reactions. It interacts with various enzymes, proteins, and other biomolecules. The nature of these interactions is largely due to its unique structural motif, which is widespread in natural products and is usually essential for biological activities .
Cellular Effects
This compound has a broad spectrum of effects on various types of cells and cellular processes. For instance, it has been found to influence cell function, including impacts on cell signaling pathways, gene expression, and cellular metabolism . These effects are likely due to the unique structural and chemical properties of this compound .
Molecular Mechanism
The molecular mechanism of action of this compound involves its unique structural properties. It exerts its effects at the molecular level through binding interactions with biomolecules, enzyme inhibition or activation, and changes in gene expression . The unique reactivity of this compound is due to its strained ring structure .
Temporal Effects in Laboratory Settings
In laboratory settings, the effects of this compound can change over time. This includes information on the product’s stability, degradation, and any long-term effects on cellular function observed in in vitro or in vivo studies .
Dosage Effects in Animal Models
The effects of this compound can vary with different dosages in animal models
Metabolic Pathways
This compound is involved in several metabolic pathways. Nature has evolved diverse strategies to access this structural motif of cyclopropane . The reactions can be grouped into two major pathways according to whether the reaction involves an exogenous C1 unit from S-adenosylmethionine (SAM) or not .
Preparation Methods
Synthetic Routes and Reaction Conditions: Cyclopropane-D6 can be synthesized through several steps starting from deuterium oxide and magnesium tricarbide. The process involves the following steps:
Methyl Acetylene-D4 Formation: Deuterium oxide reacts with magnesium tricarbide to form methyl acetylene-D4.
Propylene-D6 Formation: Methyl acetylene-D4 is then hydrogenated to form propylene-D6.
Allyl Chloride-D5 Formation: Propylene-D6 is chlorinated to form allyl chloride-D5.
3-Bromo-1-Chloropropane-D6 Formation: Allyl chloride-D5 undergoes bromination to form 3-bromo-1-chloropropane-D6.
This compound Formation: Finally, 3-bromo-1-chloropropane-D6 undergoes cyclization to form this compound.
Industrial Production Methods: While the laboratory synthesis of this compound is well-documented, industrial production methods are less common due to the specialized nature of deuterated compounds. Industrial production would likely follow similar steps but on a larger scale, with considerations for cost and efficiency.
Chemical Reactions Analysis
Types of Reactions: Cyclopropane-D6, like its non-deuterated counterpart, undergoes various chemical reactions, including:
Oxidation: this compound can be oxidized to form carbon dioxide and deuterium oxide.
Reduction: It can be reduced to form deuterated alkanes.
Substitution: this compound can undergo substitution reactions where deuterium atoms are replaced by other atoms or groups.
Common Reagents and Conditions:
Oxidation: Strong oxidizing agents such as potassium permanganate or ozone.
Reduction: Catalytic hydrogenation using deuterium gas.
Substitution: Halogenation using halogens like chlorine or bromine, followed by nucleophilic substitution.
Major Products:
Oxidation: Carbon dioxide and deuterium oxide.
Reduction: Deuterated alkanes.
Substitution: Deuterated halocyclopropanes and other substituted derivatives
Scientific Research Applications
Cyclopropane-D6 is utilized in various scientific research fields due to its unique properties:
Chemistry: Used as a model compound in studying reaction mechanisms and kinetics, especially in isotope effect studies.
Biology: Employed in metabolic studies to trace deuterium incorporation and distribution in biological systems.
Medicine: Utilized in drug design and development to study the effects of deuterium substitution on drug metabolism and efficacy.
Industry: Used in the development of deuterated materials and compounds for specialized applications, such as in nuclear magnetic resonance (NMR) spectroscopy
Comparison with Similar Compounds
Cyclopropane-D6 can be compared with other deuterated cycloalkanes and non-deuterated cyclopropane:
Cyclopropane: The non-deuterated form, C3H6, is lighter and has different vibrational spectra.
Cyclobutane-D8: Another deuterated cycloalkane with four carbon atoms and eight deuterium atoms, C4D8.
Cyclopentane-D10: A five-membered ring with ten deuterium atoms, C5D10.
Uniqueness: this compound is unique due to its three-membered ring structure and complete deuteration, which provides distinct physical and chemical properties compared to other cycloalkanes .
By understanding the properties, preparation methods, chemical reactions, and applications of this compound, researchers can leverage this compound in various scientific and industrial fields.
Biological Activity
Cyclopropane-D6 is a deuterated form of cyclopropane, which has garnered attention for its potential biological activities. This article explores the biological activity of this compound, focusing on its applications in medicinal chemistry, particularly as a structural element in drug design and its interactions with biological systems.
Structural Characteristics and Synthesis
This compound is characterized by its three-membered carbon ring structure, where hydrogen atoms are replaced by deuterium. This modification can enhance the stability and metabolic profile of compounds in which it is incorporated. The synthesis of this compound typically involves deuterated reagents, allowing for precise incorporation of deuterium into the cyclopropane framework.
Biological Activity Overview
-
Antiviral Properties :
- Cyclopropane derivatives, including this compound, have been studied for their antiviral properties, particularly against coronaviruses. Recent studies have demonstrated that cyclopropane-based inhibitors exhibit significant potency against SARS-CoV-2 and MERS-CoV.
- For instance, compounds incorporating a cyclopropyl moiety showed EC50 values as low as 11-12 nM against SARS-CoV-2 replication, indicating their potential as broad-spectrum antivirals .
-
Microtubule Dynamics :
- Research on cyclopropyl-epothilones has revealed that the configuration of the cyclopropane moiety significantly influences microtubule binding affinity and antiproliferative activity. Variants with specific stereochemistry exhibited activities comparable to natural epothilones, highlighting the importance of structural orientation in biological efficacy .
- Drug Development :
Case Study 1: Cyclopropane-Based Inhibitors Against Coronaviruses
A study focused on the design and evaluation of cyclopropane-derived inhibitors against coronavirus proteases demonstrated their high potency and low cytotoxicity. The structural modifications allowed these compounds to effectively bind to the active sites of viral enzymes, leading to significant inhibition of viral replication.
Compound | EC50 (nM) | IC50 (nM) | Safety Index |
---|---|---|---|
5c | 12 | 80 | 7692 |
11c | 11 | 120 | 9090 |
These findings suggest that cyclopropane-based compounds could serve as promising candidates for further development as direct-acting antivirals .
Case Study 2: Antiproliferative Activity of Cyclopropyl-Epothilones
In another study, two variants of cyclopropyl-epothilone B were synthesized to assess their effects on microtubule dynamics. The results indicated that one variant closely mimicked the activity of natural epothilones, while the other was significantly less effective due to stereochemical differences.
Variant | Microtubule Binding Affinity | Antiproliferative Activity |
---|---|---|
Variant A | Comparable to Epothilone A | High |
Variant B | Significantly lower | Low |
This case underscores the critical role that the cyclopropane configuration plays in determining biological activity .
Properties
IUPAC Name |
1,1,2,2,3,3-hexadeuteriocyclopropane | |
---|---|---|
Details | Computed by LexiChem 2.6.6 (PubChem release 2019.06.18) | |
Source | PubChem | |
URL | https://pubchem.ncbi.nlm.nih.gov | |
Description | Data deposited in or computed by PubChem | |
InChI |
InChI=1S/C3H6/c1-2-3-1/h1-3H2/i1D2,2D2,3D2 | |
Details | Computed by InChI 1.0.5 (PubChem release 2019.06.18) | |
Source | PubChem | |
URL | https://pubchem.ncbi.nlm.nih.gov | |
Description | Data deposited in or computed by PubChem | |
InChI Key |
LVZWSLJZHVFIQJ-NMFSSPJFSA-N | |
Details | Computed by InChI 1.0.5 (PubChem release 2019.06.18) | |
Source | PubChem | |
URL | https://pubchem.ncbi.nlm.nih.gov | |
Description | Data deposited in or computed by PubChem | |
Canonical SMILES |
C1CC1 | |
Details | Computed by OEChem 2.1.5 (PubChem release 2019.06.18) | |
Source | PubChem | |
URL | https://pubchem.ncbi.nlm.nih.gov | |
Description | Data deposited in or computed by PubChem | |
Isomeric SMILES |
[2H]C1(C(C1([2H])[2H])([2H])[2H])[2H] | |
Details | Computed by OEChem 2.1.5 (PubChem release 2019.06.18) | |
Source | PubChem | |
URL | https://pubchem.ncbi.nlm.nih.gov | |
Description | Data deposited in or computed by PubChem | |
Molecular Formula |
C3H6 | |
Details | Computed by PubChem 2.1 (PubChem release 2019.06.18) | |
Source | PubChem | |
URL | https://pubchem.ncbi.nlm.nih.gov | |
Description | Data deposited in or computed by PubChem | |
Molecular Weight |
48.12 g/mol | |
Details | Computed by PubChem 2.1 (PubChem release 2021.05.07) | |
Source | PubChem | |
URL | https://pubchem.ncbi.nlm.nih.gov | |
Description | Data deposited in or computed by PubChem | |
Retrosynthesis Analysis
AI-Powered Synthesis Planning: Our tool employs the Template_relevance Pistachio, Template_relevance Bkms_metabolic, Template_relevance Pistachio_ringbreaker, Template_relevance Reaxys, Template_relevance Reaxys_biocatalysis model, leveraging a vast database of chemical reactions to predict feasible synthetic routes.
One-Step Synthesis Focus: Specifically designed for one-step synthesis, it provides concise and direct routes for your target compounds, streamlining the synthesis process.
Accurate Predictions: Utilizing the extensive PISTACHIO, BKMS_METABOLIC, PISTACHIO_RINGBREAKER, REAXYS, REAXYS_BIOCATALYSIS database, our tool offers high-accuracy predictions, reflecting the latest in chemical research and data.
Strategy Settings
Precursor scoring | Relevance Heuristic |
---|---|
Min. plausibility | 0.01 |
Model | Template_relevance |
Template Set | Pistachio/Bkms_metabolic/Pistachio_ringbreaker/Reaxys/Reaxys_biocatalysis |
Top-N result to add to graph | 6 |
Feasible Synthetic Routes
Q1: What is the significance of studying the vibrational spectra of cyclopropane-D6?
A1: Analyzing the vibrational spectra of both cyclopropane and its deuterated analog, this compound, provides crucial insights into the molecular structure and dynamics of the cyclopropane ring. The isotopic substitution leads to shifts in vibrational frequencies, allowing for a more comprehensive assignment of vibrational modes and determination of force constants. [, , , ]
Q2: How does deuteration impact the rotational constants of cyclopropane?
A2: Deuteration significantly affects the rotational constants of cyclopropane. Studies using high-resolution Raman and infrared spectroscopy have determined the ground-state rotational constants for both cyclopropane and this compound. The values obtained allowed for a precise determination of the carbon-carbon (C-C) bond distance in the cyclopropane ring. [, , , ] For instance, a study utilizing high-resolution infrared spectroscopy on the ν6 band of this compound provided detailed information about its rotational structure. [, ]
Q3: What can be learned about the structure of cyclopropane from studying this compound?
A3: Analysis of rotational constants derived from high-resolution spectra of this compound, in conjunction with data from cyclopropane, enabled researchers to determine a C-C distance of 1.524 ± 0.014 Å. This value, combined with assumptions about C-H bond lengths, provided valuable information about the HCH bond angle within the cyclopropane molecule. []
Q4: How does deuteration affect the vibrational frequencies of cyclopropane?
A4: Deuteration causes a significant shift in vibrational frequencies, particularly for modes involving C-H bond stretching and bending. This shift is clearly observed in studies comparing the infrared and Raman spectra of cyclopropane and this compound. The observed isotopic shifts provide crucial information for assigning vibrational modes and understanding the nature of specific vibrations. [, , , ] For example, the C-D stretching bands (ν6 and ν8) in this compound were studied using high-resolution Fourier transform infrared spectroscopy, revealing details about their vibrational structure and interactions with other vibrational modes. []
Q5: How has this compound been used in kinetic isotope effect studies?
A5: this compound plays a critical role in kinetic isotope effect studies aimed at understanding the mechanisms of reactions involving cyclopropane. By comparing the reaction rates of cyclopropane and this compound, researchers can gain insights into the bond-breaking and bond-forming processes involved in the reaction mechanism. [, , ] One study investigated the kinetic isotope effects in the pyrolysis of cyclopropane and this compound, providing support for a diradical mechanism. [] Another study utilized this compound to investigate deuterium isotope effects on the rates of methylene radical insertion into C-H bonds. []
Q6: How does the study of this compound contribute to our understanding of Jahn-Teller distortion?
A6: this compound has been instrumental in exploring the complex dynamics of Jahn-Teller distortion in the excited state of cyclopropane. Two-photon absorption spectra of jet-cooled cyclopropane and this compound revealed vibrational activity in both ring deformation and CH2 wagging modes, indicative of Jahn-Teller distortion in the 3sE’ excited state. [] These findings provide valuable information about the interplay between electronic structure and molecular geometry in this strained ring system.
Q7: What insights into crystal structure can be obtained from studying this compound?
A7: Analysis of infrared and Raman spectra of crystalline this compound, along with mixed crystals of cyclopropane and this compound, has been crucial in understanding the nature of multiplet splittings in their spectra. These studies, coupled with x-ray diffraction data, suggest an orthorhombic unit cell for crystalline cyclopropane and provide insights into the crystal structure and intermolecular interactions in the solid state. []
Q8: What makes this compound useful in studying energy transfer processes?
A8: this compound has been used in investigations of gas-surface vibrational energy transfer. The distinct vibrational frequencies of this compound, compared to cyclopropane, allow researchers to track energy transfer processes between the gas-phase molecule and a solid surface. [] These studies provide valuable insights into the fundamental processes governing energy exchange at the gas-solid interface.
Disclaimer and Information on In-Vitro Research Products
Please be aware that all articles and product information presented on BenchChem are intended solely for informational purposes. The products available for purchase on BenchChem are specifically designed for in-vitro studies, which are conducted outside of living organisms. In-vitro studies, derived from the Latin term "in glass," involve experiments performed in controlled laboratory settings using cells or tissues. It is important to note that these products are not categorized as medicines or drugs, and they have not received approval from the FDA for the prevention, treatment, or cure of any medical condition, ailment, or disease. We must emphasize that any form of bodily introduction of these products into humans or animals is strictly prohibited by law. It is essential to adhere to these guidelines to ensure compliance with legal and ethical standards in research and experimentation.