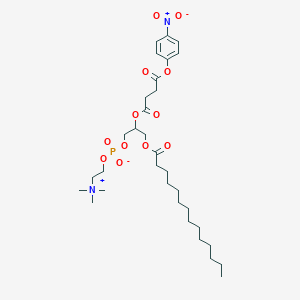
sn-Glycero-3-phosphocholine A
Overview
Description
Mechanism of Action
Target of Action
The primary target of sn-Glycero-3-phosphocholine A, also known as 14:0 NPS PC, is the lipoprotein-associated phospholipase A2 . This enzyme plays a crucial role in the metabolism of phosphatidylcholine, a primary component of biological membranes .
Mode of Action
The compound interacts with its target by associating with lipids or detergents, which is essential for preserving the active structure of lipoprotein-associated phospholipase A2 . This interaction leads to an increase in acetylcholine synthesis and release in the brain .
Biochemical Pathways
This compound is a precursor to choline biosynthesis and an intermediate in the metabolism of phosphatidylcholine . In vivo, it is converted to phosphorylcholine, a metabolically active form of choline . This conversion affects the cholinergic neurotransmission pathway, leading to increased synthesis and release of acetylcholine, a key neurotransmitter in the brain .
Pharmacokinetics
It is known that this compound can rapidly deliver choline to the brain across the blood-brain barrier , suggesting good bioavailability.
Result of Action
The action of this compound leads to increased acetylcholine synthesis and release in the brain . This can enhance cognitive function and has potential implications for the treatment of conditions like Alzheimer’s disease and dementia .
Action Environment
It is soluble in ethanol, dmso, and a mixture of chloroform, methanol, and water , suggesting that its action might be influenced by the solvent environment.
Biochemical Analysis
Biochemical Properties
sn-Glycero-3-phosphocholine A plays a significant role in biochemical reactions. It rapidly delivers choline to the brain across the blood–brain barrier and is a biosynthetic precursor of the acetylcholine neurotransmitter . It interacts with various enzymes, proteins, and other biomolecules. For instance, it is synthesized from sn-glycero-3-phosphocholine using Steglich esterification .
Cellular Effects
This compound has profound effects on various types of cells and cellular processes. It influences cell function by increasing acetylcholine synthesis and release in rat hippocampus . It also has an impact on cell signaling pathways, gene expression, and cellular metabolism .
Molecular Mechanism
The molecular mechanism of this compound involves its conversion to phosphorylcholine, a metabolically active form of choline that increases acetylcholine synthesis and release in rat hippocampus . This process involves binding interactions with biomolecules, enzyme inhibition or activation, and changes in gene expression .
Temporal Effects in Laboratory Settings
In laboratory settings, the effects of this compound change over time. It has been observed that DMPC/PVP-SPIONs, which are coated with this compound, were effectively distributed on the membranes of axons, as well as dendritic and myelin sheaths . This suggests that this compound has long-term effects on cellular function.
Metabolic Pathways
This compound is involved in several metabolic pathways. It is synthesized by two distinct pathways . The de novo pathway utilizes 1-alkyl-2-acetyl-sn-glycerol and CDP-choline as substrates of a DTT-insensitive phosphocholine transferase . The remodeling pathway requires the production of 1-alkyl-2-lyso-sn-glycero-3-phosphocholine .
Transport and Distribution
This compound is transported and distributed within cells and tissues. It rapidly delivers choline to the brain across the blood–brain barrier . It is also distributed on the membranes of axons, dendritic and myelin sheaths .
Subcellular Localization
The subcellular localization of this compound is primarily in the brain . It is found in the plasma membrane and the microsomal fraction
Preparation Methods
Synthetic Routes and Reaction Conditions: sn-Glycero-3-phosphocholine A can be synthesized through the chemical or enzymatic deacylation of phosphatidylcholine enriched soya phospholipids followed by chromatographic purification . Another method involves the use of sn-glycero-3-phosphocholine-kieselguhr complex in a solvent-free environment, which provides high yields and allows for the recycling of kieselguhr .
Industrial Production Methods: Industrially, this compound is produced by the chemical or enzymatic deacylation of phosphatidylcholine enriched soya phospholipids followed by chromatographic purification . It can also be derived in small amounts from highly purified soy lecithin and sunflower lecithin .
Chemical Reactions Analysis
Types of Reactions: sn-Glycero-3-phosphocholine A undergoes various chemical reactions, including hydrolysis, oxidation, and substitution reactions. For instance, it hydrolyzes to give oleic acid and several phosphorus-containing products when subjected to high-temperature water .
Common Reagents and Conditions:
Hydrolysis: Catalyzed by oleic and phosphoric acids.
Oxidation: Involves the use of oxidizing agents like hydrogen peroxide.
Substitution: Utilizes reagents such as alkyl halides.
Major Products Formed:
Hydrolysis: Oleic acid and phosphorus-containing products.
Oxidation: Various oxidized derivatives of this compound.
Substitution: Alkylated derivatives of the compound.
Scientific Research Applications
sn-Glycero-3-phosphocholine A has a wide range of scientific research applications:
Chemistry: Used as a precursor in the synthesis of other phospholipids and choline-containing compounds.
Biology: Studied for its role in cell membrane structure and function.
Medicine: Investigated for its potential in treating cognitive impairments, Alzheimer’s disease, and other dementias.
Industry: Utilized as an emulsifier in bioactive delivery systems and food industry applications.
Comparison with Similar Compounds
1,2-Dimyristoyl-sn-glycero-3-phosphocholine (DMPC): Used in neurotherapy and drug delivery.
1,2-Dioleoyl-sn-glycero-3-phosphocholine (DOPC): Utilized in the preparation of graphene suspensions.
1,2-Distearoyl-sn-glycero-3-phosphocholine (DSPC): Employed in the formation of lipid nanoparticles.
Uniqueness: sn-Glycero-3-phosphocholine A is unique due to its rapid delivery of choline to the brain and its role as a biosynthetic precursor of acetylcholine . This makes it particularly valuable in the treatment of cognitive impairments and neurodegenerative diseases.
Properties
IUPAC Name |
[2-[4-(4-nitrophenoxy)-4-oxobutanoyl]oxy-3-tetradecanoyloxypropyl] 2-(trimethylazaniumyl)ethyl phosphate | |
---|---|---|
Details | Computed by LexiChem 2.6.6 (PubChem release 2019.06.18) | |
Source | PubChem | |
URL | https://pubchem.ncbi.nlm.nih.gov | |
Description | Data deposited in or computed by PubChem | |
InChI |
InChI=1S/C32H53N2O12P/c1-5-6-7-8-9-10-11-12-13-14-15-16-30(35)42-25-29(26-44-47(40,41)43-24-23-34(2,3)4)46-32(37)22-21-31(36)45-28-19-17-27(18-20-28)33(38)39/h17-20,29H,5-16,21-26H2,1-4H3 | |
Details | Computed by InChI 1.0.5 (PubChem release 2019.06.18) | |
Source | PubChem | |
URL | https://pubchem.ncbi.nlm.nih.gov | |
Description | Data deposited in or computed by PubChem | |
InChI Key |
XOCHZRRLIIQGMZ-UHFFFAOYSA-N | |
Details | Computed by InChI 1.0.5 (PubChem release 2019.06.18) | |
Source | PubChem | |
URL | https://pubchem.ncbi.nlm.nih.gov | |
Description | Data deposited in or computed by PubChem | |
Canonical SMILES |
CCCCCCCCCCCCCC(=O)OCC(COP(=O)([O-])OCC[N+](C)(C)C)OC(=O)CCC(=O)OC1=CC=C(C=C1)[N+](=O)[O-] | |
Details | Computed by OEChem 2.1.5 (PubChem release 2019.06.18) | |
Source | PubChem | |
URL | https://pubchem.ncbi.nlm.nih.gov | |
Description | Data deposited in or computed by PubChem | |
Molecular Formula |
C32H53N2O12P | |
Details | Computed by PubChem 2.1 (PubChem release 2019.06.18) | |
Source | PubChem | |
URL | https://pubchem.ncbi.nlm.nih.gov | |
Description | Data deposited in or computed by PubChem | |
Molecular Weight |
688.7 g/mol | |
Details | Computed by PubChem 2.1 (PubChem release 2021.05.07) | |
Source | PubChem | |
URL | https://pubchem.ncbi.nlm.nih.gov | |
Description | Data deposited in or computed by PubChem | |
Retrosynthesis Analysis
AI-Powered Synthesis Planning: Our tool employs the Template_relevance Pistachio, Template_relevance Bkms_metabolic, Template_relevance Pistachio_ringbreaker, Template_relevance Reaxys, Template_relevance Reaxys_biocatalysis model, leveraging a vast database of chemical reactions to predict feasible synthetic routes.
One-Step Synthesis Focus: Specifically designed for one-step synthesis, it provides concise and direct routes for your target compounds, streamlining the synthesis process.
Accurate Predictions: Utilizing the extensive PISTACHIO, BKMS_METABOLIC, PISTACHIO_RINGBREAKER, REAXYS, REAXYS_BIOCATALYSIS database, our tool offers high-accuracy predictions, reflecting the latest in chemical research and data.
Strategy Settings
Precursor scoring | Relevance Heuristic |
---|---|
Min. plausibility | 0.01 |
Model | Template_relevance |
Template Set | Pistachio/Bkms_metabolic/Pistachio_ringbreaker/Reaxys/Reaxys_biocatalysis |
Top-N result to add to graph | 6 |
Feasible Synthetic Routes
Q1: What is the role of cholesterol in phospholipid membranes?
A: Cholesterol plays a crucial chemoprotective role in lipid membranes. Studies using field-induced droplet ionization mass spectrometry revealed that adding 30 mol% cholesterol to 1,2-dipalmitoyl-sn-glycero-3-phosphocholine (DPPC) or 1-palmitoyl-2-oleoyl-sn-glycero-3-phospho-(1′-rac-glycerol) (POPG) monolayers significantly reduced oxidation and ester cleavage caused by reactive species like hydroxyl radicals and HCl vapor. This protection is attributed to cholesterol's ability to increase lipid packing density and alter membrane fluidity. []
Q2: How does the presence of double bonds in polyunsaturated fatty acids affect membrane properties?
A: Double bonds in polyunsaturated fatty acids significantly impact membrane fluidity and interactions with other lipids. Research comparing membranes containing docosahexaenoic acid (DHA, 22:6n3) and docosapentaenoic acid (DPA, 22:5n6) revealed that the additional double bond in DHA increased its flexibility at the methyl end, leading to shorter isomerization correlation times. This difference in flexibility also influences the packing of saturated fatty acids within the membrane. []
Q3: How does the structure of amphipathic α-helical peptides influence their interaction with phospholipids?
A: The structure of amphipathic α-helical peptides directly impacts their ability to form discoidal phospholipid complexes called nanodiscs. Comparing nanodiscs formed by the peptide 18A and apolipoprotein A-I (apoA-I) revealed key differences. While 18A nanodiscs maintain a planar lipid bilayer irrespective of size, apoA-I nanodiscs exhibit size-dependent bilayer deformation into a saddle surface. This difference is attributed to the flexible helix chain structure of apoA-I. []
Q4: What role does acetyl-CoA:1-O-alkyl-2-lyso-sn-glycero-3-phosphocholine acetyltransferase play in inflammation?
A: Acetyl-CoA:1-O-alkyl-2-lyso-sn-glycero-3-phosphocholine acetyltransferase is a key enzyme in the biosynthesis of platelet-activating factor (PAF) via the remodeling pathway. Studies in human neutrophils demonstrated that this enzyme is directly activated by p38 kinase, a mitogen-activated protein kinase. Stimuli like tumor necrosis factor-α (TNF-α) that activate p38 kinase increased acetyltransferase activity, while inhibitors of p38 kinase abolished this activation. This suggests a critical role for p38 kinase-mediated acetyltransferase activation in inflammation. []
Q5: How do cholesterol levels affect phospholipid repair mechanisms in red blood cells?
A: Elevated cholesterol levels in red blood cell membranes inhibit phospholipid repair mechanisms. Studies show that cholesterol-loading decreases the incorporation of arachidonate into phospholipids and increases its uptake into acylcarnitine, a fatty acid repair intermediate. This inhibition is linked to decreased activity of arachidonoyl-CoA:l-palmitoyl-sn-glycero-3-phosphocholine acyl transferase (LAT), a key enzyme in the phospholipid repair pathway. []
Q6: Is there a difference in the synthesis of PAF and 1-acyl-2-acetyl-sn-glycero-3-phosphocholine (1-acyl-2-acetyl-GPC) by different human inflammatory cells?
A: Yes, human inflammatory cells exhibit cell-type specific differences in the synthesis of PAF and 1-acyl-2-acetyl-GPC. While most inflammatory cells, except platelets, can synthesize both molecules, some cells like mast cells, basophils, and endothelial cells predominantly produce 1-acyl-2-acetyl-GPC. In contrast, neutrophils, eosinophils, and lung macrophages primarily synthesize PAF. This suggests distinct roles for these two phospholipids in various inflammatory processes. []
Q7: What are the advantages of using phospholipid-detergent conjugates for gene delivery?
A: Phospholipid-detergent conjugates offer advantages as fusogenic carriers for gene delivery. Studies using 1,2-dioleoyl-sn-glycero-3-phosphocholine (DOPC) conjugated with detergent C12E4 demonstrated efficient DNA delivery to cells with minimal toxicity. The fusogenic properties, crucial for intracellular delivery, were directly correlated with the length of the detergent's polyether moiety. In vivo experiments showed DOPC-C12E4's high potency for lung gene delivery, making it a promising alternative to existing methods. []
Q8: How can mass spectrometry be utilized to study phospholipid oxidation?
A: Field-induced droplet ionization mass spectrometry (FIDI-MS) enables the direct measurement of chemical degradation in phospholipid monolayers at the air-water interface. By exposing DPPC and POPG monolayers to reactive species like OH radicals and HCl vapor, FIDI-MS allows researchers to quantify the extent of lipid oxidation and identify specific reaction products, providing insights into the chemoprotective role of cholesterol in these systems. []
Q9: What is the role of ammonium salts in electrospray ionization mass spectrometry of phosphatidylcholines?
A: While ammonium salts like ammonium acetate are commonly added to the mobile phase during electrospray ionization mass spectrometry of phosphatidylcholines (PCs), their role is not to directly protonate the PC molecule. Instead, experiments using deuterated ammonium acetate demonstrated that water acts as the proton source, forming [M + D]+ ions. Adding ammonium salts or organic acids can even suppress PC ionization in some cases. []
Disclaimer and Information on In-Vitro Research Products
Please be aware that all articles and product information presented on BenchChem are intended solely for informational purposes. The products available for purchase on BenchChem are specifically designed for in-vitro studies, which are conducted outside of living organisms. In-vitro studies, derived from the Latin term "in glass," involve experiments performed in controlled laboratory settings using cells or tissues. It is important to note that these products are not categorized as medicines or drugs, and they have not received approval from the FDA for the prevention, treatment, or cure of any medical condition, ailment, or disease. We must emphasize that any form of bodily introduction of these products into humans or animals is strictly prohibited by law. It is essential to adhere to these guidelines to ensure compliance with legal and ethical standards in research and experimentation.