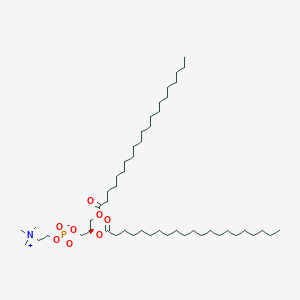
1,2-Diheneicosanoyl-sn-glycero-3-phosphocholine
Overview
Description
1,2-Diheneicosanoyl-sn-glycero-3-phosphocholine (abbreviated as 21:0PC) is a synthetic phosphatidylcholine (PC) featuring two fully saturated heneicosanoyl (21-carbon) acyl chains esterified to the sn-1 and sn-2 positions of the glycerol backbone. This phospholipid is notable for its long hydrocarbon chains, which confer unique biophysical properties, including high phase transition temperatures and enhanced membrane rigidity. It is frequently employed in liposomal formulations for drug delivery and as an internal standard in lipidomic analyses due to its stability and low natural abundance in biological systems .
Preparation Methods
Synthetic Routes and Reaction Conditions
1,2-Diheneicosanoyl-sn-glycero-3-phosphocholine can be synthesized through a multi-step process involving the esterification of glycerol with heneicosanoic acid. The synthetic route typically involves the following steps:
Esterification: Glycerol is esterified with heneicosanoic acid in the presence of a catalyst such as sulfuric acid or p-toluenesulfonic acid. The reaction is carried out under reflux conditions to form 1,2-diheneicosanoyl-sn-glycerol.
Phosphorylation: The diesterified glycerol is then phosphorylated using phosphorus oxychloride (POCl3) or phosphoric acid to introduce the phosphocholine headgroup.
Purification: The final product is purified using techniques such as column chromatography or recrystallization to obtain high-purity this compound.
Industrial Production Methods
Industrial production of this compound involves large-scale synthesis using similar reaction conditions as described above. The process is optimized for higher yields and purity, often involving automated systems for precise control of reaction parameters. The use of high-performance liquid chromatography (HPLC) and other analytical techniques ensures the quality and consistency of the final product.
Chemical Reactions Analysis
Types of Reactions
1,2-Diheneicosanoyl-sn-glycero-3-phosphocholine undergoes various chemical reactions, including:
Oxidation: The fatty acid chains can undergo oxidation reactions, leading to the formation of hydroperoxides and other oxidative degradation products.
Hydrolysis: The ester bonds in the molecule can be hydrolyzed under acidic or basic conditions, resulting in the release of free fatty acids and glycerophosphocholine.
Substitution: The phosphocholine headgroup can participate in substitution reactions, where the choline moiety is replaced by other functional groups.
Common Reagents and Conditions
Oxidation: Common oxidizing agents include hydrogen peroxide (H2O2) and potassium permanganate (KMnO4). The reactions are typically carried out under mild conditions to prevent extensive degradation.
Hydrolysis: Acidic hydrolysis can be performed using hydrochloric acid (HCl), while basic hydrolysis can be carried out using sodium hydroxide (NaOH).
Substitution: Substitution reactions often involve nucleophiles such as amines or thiols, and are carried out under controlled pH conditions to ensure selectivity.
Major Products Formed
Oxidation: Hydroperoxides, aldehydes, and ketones.
Hydrolysis: Free fatty acids and glycerophosphocholine.
Substitution: Modified phosphocholine derivatives with different headgroups.
Scientific Research Applications
Biochemical Research
Surfactant Properties and Antibacterial Activity
- 21:0 PC exhibits surfactant properties, making it effective in stabilizing emulsions and enhancing the solubility of hydrophobic compounds. Its antibacterial activity has been documented, indicating its potential in combating infections by preventing pathogen spread in biological systems .
Role in Lipid Bilayers
- As a component of lipid bilayers, this compound contributes to membrane fluidity and integrity. Its amphipathic nature allows it to form stable bilayers, essential for cell membrane structure and function .
Drug Delivery Systems
Liposome Formation
- 21:0 PC is utilized in the formulation of liposomes, which are spherical vesicles composed of lipid bilayers. These liposomes serve as carriers for drug delivery, enhancing the bioavailability and targeted delivery of therapeutic agents . The ability to encapsulate both hydrophilic and hydrophobic drugs makes liposomes versatile tools in pharmacology.
Gene Therapy Applications
- In gene therapy, this compound is employed to facilitate the delivery of nucleic acids into cells. Its role in forming lipoplexes allows for efficient transfection, making it valuable for therapeutic interventions targeting genetic disorders.
Immunological Studies
Molecular Tools in Immunology
- The compound has been explored as a molecular tool in various immunological applications. Its interactions with immune cells can provide insights into the mechanisms of immune response and inflammation .
Vaccine Development
- In vaccine research, phospholipids like 21:0 PC are investigated for their ability to enhance the immunogenicity of antigens when used as adjuvants. This application is crucial for developing more effective vaccines against infectious diseases .
Case Studies
Mechanism of Action
1,2-Diheneicosanoyl-sn-glycero-3-phosphocholine exerts its effects primarily through its incorporation into lipid bilayers. The long-chain fatty acids contribute to the stability and fluidity of the membrane, while the phosphocholine headgroup interacts with other membrane components. The compound can modulate membrane properties, such as permeability and phase behavior, and influence the activity of membrane-associated proteins and enzymes. The molecular targets and pathways involved include:
Membrane Proteins: Interaction with ion channels, receptors, and transporters.
Signaling Pathways: Modulation of signaling cascades involving phosphatidylinositol 4,5-bisphosphate (PIP2) and other phospholipids.
Comparison with Similar Compounds
Comparison with Similar Phosphatidylcholines
Structural and Biophysical Properties
Phosphatidylcholines vary primarily in acyl chain length, saturation, and molecular packing. Below is a comparative analysis of 21:0PC with structurally analogous PCs:
Table 1: Structural and Biophysical Comparison
Key Observations:
Chain Length and Phase Behavior :
- Longer saturated acyl chains (e.g., 21:0PC) increase hydrophobic interactions, elevating phase transition temperatures. For instance, DMPC (14:0) transitions at ~23°C, while 21:0PC is estimated to transition near 70°C, maintaining a gel phase at physiological temperatures. This property makes 21:0PC ideal for applications requiring low membrane permeability, such as α-particle emitter retention in liposomes .
- Unsaturated PCs like DOPC (18:1) exhibit fluid bilayers even at subzero temperatures due to kinked acyl chains, which disrupt packing .
Molecular Weight and Applications :
- Heavier PCs (e.g., 21:0PC, 930.41 g/mol) are less common in biological systems, making them suitable internal standards for lipid quantification via HPLC-MS .
- Medium-chain PCs like DPPC (16:0) are widely used in pulmonary surfactant models due to their intermediate phase behavior (~41°C) .
Functional and Practical Differences
Liposomal Stability and Drug Delivery :
- 21:0PC : Forms highly rigid bilayers, reducing drug leakage and enhancing retention of therapeutic agents (e.g., actinium-225 decay products) .
- DSPC (18:0) : Used in temperature-triggered drug release systems due to its sharp phase transition (~55°C), but less stable than 21:0PC at physiological temperatures .
- DOPC (18:1) : Preferred for mimicking fluid cellular membranes but unsuitable for long-term storage due to high permeability .
Analytical Utility :
- 21:0PC’s unique chain length minimizes interference in lipidomics, unlike naturally abundant PCs (e.g., DPPC in lung surfactant) .
Biological Activity
1,2-Diheneicosanoyl-sn-glycero-3-phosphocholine (21:0 PC) is a phospholipid belonging to the phosphatidylcholine class, characterized by two heneicosanoyl fatty acid chains and a phosphocholine head group. This unique structure contributes significantly to its biological activity, particularly in cellular processes and membrane dynamics.
Chemical Structure and Properties
This compound features:
- Fatty Acid Chains : Two long-chain fatty acids (21 carbons each).
- Amphipathic Nature : The hydrophilic head group and hydrophobic tails facilitate the formation of lipid bilayers essential for cell membranes.
This compound exhibits a high liquid-to-gel phase transition temperature, which influences its bioavailability and interactions within biological systems .
Cell Membrane Dynamics
This compound plays a crucial role in maintaining the structural integrity and fluidity of cell membranes. Its incorporation into lipid bilayers enhances membrane stability and influences membrane protein function.
Cell Signaling
The compound is involved in various cellular signaling pathways. It participates in the transfer of phosphatidylinositol and phosphatidylcholine between membranes, which is critical for signal transduction processes .
Drug Delivery Systems
Due to its ability to form liposomes, this compound is extensively used in drug delivery applications. Liposomes created with this phospholipid can encapsulate therapeutic agents, facilitating targeted delivery and improving bioavailability .
Study on Liposome Formation
A study investigated the use of this compound in liposome formulations for drug delivery. The results indicated that liposomes formed with this compound exhibited enhanced stability and encapsulation efficiency compared to those made with shorter-chain phospholipids. This stability makes it suitable for delivering sensitive therapeutic agents .
Antibacterial Activity
Research has shown that this compound possesses antibacterial properties. In vitro studies demonstrated its effectiveness against various bacterial strains, suggesting potential applications in developing antimicrobial agents or preservatives in pharmaceuticals and cosmetics .
Biochemical Pathways Involved
This compound is synthesized through two main pathways:
- De Novo Pathway : Involves the synthesis of phospholipids from simpler molecules.
- Remodeling Pathway : Involves the modification of existing phospholipids to maintain membrane composition under varying physiological conditions .
Table of Biological Activities
Q & A
Basic Research Questions
Q. What are the standard synthetic routes for preparing 1,2-diheneicosanoyl-sn-glycero-3-phosphocholine, and how do reaction conditions influence acyl chain homogeneity?
- The synthesis typically involves acylation of sn-glycero-3-phosphocholine (GPC) with heneicosanoyl chloride derivatives. A two-step protocol is often employed: (1) activation of fatty acids (e.g., via anhydride or chloride formation) and (2) regioselective esterification under controlled anhydrous conditions . Temperature and solvent polarity critically impact acyl chain homogeneity. For instance, polar aprotic solvents (e.g., chloroform) at 40–50°C minimize side reactions like acyl migration, ensuring >95% purity .
Q. How is the purity and structural integrity of this compound validated in lipidomics studies?
- High-performance liquid chromatography (HPLC) coupled with evaporative light scattering detection (ELSD) or mass spectrometry (MS) is standard. Retention time alignment with synthetic standards and molecular ion peaks (e.g., [M+H]⁺ at m/z 789.6) confirm identity . Nuclear magnetic resonance (NMR) spectroscopy, particularly ¹H and ³¹P NMR, resolves stereochemical integrity at the sn-3 position and detects trace lyso-derivatives .
Q. What solvent systems are optimal for reconstituting this compound in model membrane studies?
- Chloroform:methanol (2:1, v/v) is preferred for initial dissolution. For aqueous dispersion (e.g., liposome formation), ethanol injection or thin-film hydration with Tris-HCl buffer (pH 7.4) achieves homogeneous unilamellar vesicles. Critical micellar concentration (CMC) must be determined via fluorescent probes (e.g., diphenylhexatriene) to avoid aggregation artifacts .
Q. What safety protocols are essential when handling this compound in the laboratory?
- While not classified as acutely toxic, prolonged exposure to aerosols requires PPE (gloves, goggles, respirators). Storage at −20°C under argon prevents oxidation. Spills should be neutralized with inert absorbents (e.g., vermiculite) and disposed via hazardous waste channels .
Advanced Research Questions
Q. How does the acyl chain length of this compound influence bilayer rigidity in molecular dynamics simulations?
- The C21:0 acyl chains increase membrane thickness (~5.2 nm) and reduce lateral diffusion coefficients (Dₗ ~1.5 × 10⁻⁸ cm²/s) compared to shorter-chain analogs (e.g., C16:0). Coarse-grained simulations reveal enhanced van der Waals interactions between saturated chains, stabilizing lipid rafts in heterogeneous membranes .
Q. What metabolic pathways degrade this compound in cellular systems, and how do lysophosphatidylcholines (lyso-PCs) affect inflammatory signaling?
- Phospholipase A₂ (PLA₂) hydrolyzes the sn-2 acyl chain, generating 1-heneicosanoyl-lyso-PC. In monocytes, lyso-PC derivatives at 12.5 µM upregulate IL-1β via NLRP3 inflammasome activation, confirmed by caspase-1 inhibition assays. Paradoxically, cancer patient plasma shows reduced lyso-PC levels, suggesting context-dependent roles in inflammation .
Q. How do discrepancies in reported phase transition temperatures (Tₘ) for this compound arise, and how can experimental design mitigate them?
- Tₘ values vary due to hydration levels and heating rates in differential scanning calorimetry (DSC). Fully hydrated bilayers exhibit Tₘ ~55°C, while anhydrous samples show Tₘ >70°C. Pre-equilibration in deuterated water for 24 h and slow scanning (1°C/min) improve reproducibility .
Q. What isotopic labeling strategies enable tracking of this compound in metabolic flux analysis?
- Deuterium labeling at acyl chain termini (e.g., d₄₄-heneicosanoic acid) or ¹³C-enriched choline headgroups permits LC-MS/MS quantification in lipid turnover studies. Stable isotope tracer kinetics (SITK) models resolve incorporation rates into cellular membranes .
Q. How can molecular docking studies predict interactions between this compound and transmembrane proteins (e.g., GPCRs)?
- All-atom force fields (e.g., CHARMM36) simulate lipid-protein binding pockets. Free energy perturbation (FEP) calculations reveal preferential binding of C21:0 chains to hydrophobic helices, modulating receptor conformational states. Validation via surface plasmon resonance (SPR) shows KD ~10⁻⁶ M for β₂-adrenergic receptors .
Q. Methodological Considerations Table
Properties
IUPAC Name |
[(2R)-2,3-di(henicosanoyloxy)propyl] 2-(trimethylazaniumyl)ethyl phosphate | |
---|---|---|
Details | Computed by LexiChem 2.6.6 (PubChem release 2019.06.18) | |
Source | PubChem | |
URL | https://pubchem.ncbi.nlm.nih.gov | |
Description | Data deposited in or computed by PubChem | |
InChI |
InChI=1S/C50H100NO8P/c1-6-8-10-12-14-16-18-20-22-24-26-28-30-32-34-36-38-40-42-49(52)56-46-48(47-58-60(54,55)57-45-44-51(3,4)5)59-50(53)43-41-39-37-35-33-31-29-27-25-23-21-19-17-15-13-11-9-7-2/h48H,6-47H2,1-5H3/t48-/m1/s1 | |
Details | Computed by InChI 1.0.5 (PubChem release 2019.06.18) | |
Source | PubChem | |
URL | https://pubchem.ncbi.nlm.nih.gov | |
Description | Data deposited in or computed by PubChem | |
InChI Key |
QFFSGJSMHPWZOB-QSCHNALKSA-N | |
Details | Computed by InChI 1.0.5 (PubChem release 2019.06.18) | |
Source | PubChem | |
URL | https://pubchem.ncbi.nlm.nih.gov | |
Description | Data deposited in or computed by PubChem | |
Canonical SMILES |
CCCCCCCCCCCCCCCCCCCCC(=O)OCC(COP(=O)([O-])OCC[N+](C)(C)C)OC(=O)CCCCCCCCCCCCCCCCCCCC | |
Details | Computed by OEChem 2.1.5 (PubChem release 2019.06.18) | |
Source | PubChem | |
URL | https://pubchem.ncbi.nlm.nih.gov | |
Description | Data deposited in or computed by PubChem | |
Isomeric SMILES |
CCCCCCCCCCCCCCCCCCCCC(=O)OC[C@H](COP(=O)([O-])OCC[N+](C)(C)C)OC(=O)CCCCCCCCCCCCCCCCCCCC | |
Details | Computed by OEChem 2.1.5 (PubChem release 2019.06.18) | |
Source | PubChem | |
URL | https://pubchem.ncbi.nlm.nih.gov | |
Description | Data deposited in or computed by PubChem | |
Molecular Formula |
C50H100NO8P | |
Details | Computed by PubChem 2.1 (PubChem release 2019.06.18) | |
Source | PubChem | |
URL | https://pubchem.ncbi.nlm.nih.gov | |
Description | Data deposited in or computed by PubChem | |
Molecular Weight |
874.3 g/mol | |
Details | Computed by PubChem 2.1 (PubChem release 2021.05.07) | |
Source | PubChem | |
URL | https://pubchem.ncbi.nlm.nih.gov | |
Description | Data deposited in or computed by PubChem | |
Retrosynthesis Analysis
AI-Powered Synthesis Planning: Our tool employs the Template_relevance Pistachio, Template_relevance Bkms_metabolic, Template_relevance Pistachio_ringbreaker, Template_relevance Reaxys, Template_relevance Reaxys_biocatalysis model, leveraging a vast database of chemical reactions to predict feasible synthetic routes.
One-Step Synthesis Focus: Specifically designed for one-step synthesis, it provides concise and direct routes for your target compounds, streamlining the synthesis process.
Accurate Predictions: Utilizing the extensive PISTACHIO, BKMS_METABOLIC, PISTACHIO_RINGBREAKER, REAXYS, REAXYS_BIOCATALYSIS database, our tool offers high-accuracy predictions, reflecting the latest in chemical research and data.
Strategy Settings
Precursor scoring | Relevance Heuristic |
---|---|
Min. plausibility | 0.01 |
Model | Template_relevance |
Template Set | Pistachio/Bkms_metabolic/Pistachio_ringbreaker/Reaxys/Reaxys_biocatalysis |
Top-N result to add to graph | 6 |
Feasible Synthetic Routes
Disclaimer and Information on In-Vitro Research Products
Please be aware that all articles and product information presented on BenchChem are intended solely for informational purposes. The products available for purchase on BenchChem are specifically designed for in-vitro studies, which are conducted outside of living organisms. In-vitro studies, derived from the Latin term "in glass," involve experiments performed in controlled laboratory settings using cells or tissues. It is important to note that these products are not categorized as medicines or drugs, and they have not received approval from the FDA for the prevention, treatment, or cure of any medical condition, ailment, or disease. We must emphasize that any form of bodily introduction of these products into humans or animals is strictly prohibited by law. It is essential to adhere to these guidelines to ensure compliance with legal and ethical standards in research and experimentation.