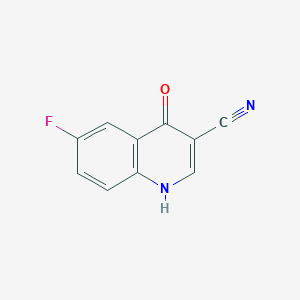
6-Fluoro-4-oxo-1,4-dihydroquinoline-3-carbonitrile
Overview
Description
6-Fluoro-4-oxo-1,4-dihydroquinoline-3-carbonitrile is a member of the fluoroquinolone family, which is known for its broad-spectrum antibacterial properties. This compound is characterized by the presence of a fluorine atom at the 6th position, a carbonyl group at the 4th position, and a nitrile group at the 3rd position of the quinoline ring. The incorporation of these functional groups enhances its biological activity and makes it a valuable compound in medicinal chemistry .
Mechanism of Action
Target of Action
The primary targets of 6-Fluoro-4-oxo-1,4-dihydroquinoline-3-carbonitrile are bacterial type II topoisomerase enzymes, specifically DNA gyrase and topoisomerase IV . These enzymes are essential for bacterial DNA replication, transcription, repair, and recombination .
Mode of Action
This compound interacts with its targets by selectively inhibiting these enzymes . This inhibition blocks the untwisting process required to replicate one DNA double helix into two . This interaction results in the prevention of bacterial DNA replication, leading to the death of the bacteria .
Biochemical Pathways
The compound affects the DNA replication pathway in bacteria. By inhibiting DNA gyrase and topoisomerase IV, it prevents the unwinding of the DNA helix, a crucial step in DNA replication . This disruption leads to the cessation of bacterial growth and multiplication .
Pharmacokinetics
Fluoroquinolones, a class of compounds to which this compound belongs, are generally well absorbed from the gastrointestinal tract, widely distributed in body tissues, metabolized in the liver, and excreted via the kidneys .
Result of Action
The molecular effect of this compound’s action is the inhibition of bacterial DNA replication . On a cellular level, this results in the prevention of bacterial growth and multiplication, leading to the death of the bacteria .
Preparation Methods
Synthetic Routes and Reaction Conditions
The synthesis of 6-Fluoro-4-oxo-1,4-dihydroquinoline-3-carbonitrile typically involves the following steps:
Starting Material: The synthesis begins with the preparation of 2,4-dichloro-5-fluorobenzoylacetate.
Cyclization: The intermediate undergoes cyclization to form the quinoline ring.
Functional Group Introduction: The nitrile group is introduced at the 3rd position, and the carbonyl group is introduced at the 4th position through various chemical reactions.
Industrial Production Methods
Industrial production of this compound often involves multi-step synthesis processes that are optimized for high yield and purity. These processes may include the use of catalysts, controlled reaction conditions, and purification techniques to ensure the final product meets the required specifications .
Chemical Reactions Analysis
Types of Reactions
6-Fluoro-4-oxo-1,4-dihydroquinoline-3-carbonitrile undergoes several types of chemical reactions, including:
Oxidation: The compound can be oxidized to introduce additional functional groups.
Reduction: Reduction reactions can modify the carbonyl group to form alcohols or other derivatives.
Substitution: The fluorine atom can be substituted with other groups to create derivatives with different properties.
Common Reagents and Conditions
Oxidation: Common oxidizing agents include potassium permanganate and chromium trioxide.
Reduction: Reducing agents such as sodium borohydride and lithium aluminum hydride are used.
Substitution: Nucleophilic substitution reactions often involve reagents like sodium methoxide or potassium tert-butoxide.
Major Products
The major products formed from these reactions include various quinoline derivatives with modified functional groups, which can exhibit different biological activities and properties .
Scientific Research Applications
6-Fluoro-4-oxo-1,4-dihydroquinoline-3-carbonitrile has several scientific research applications:
Chemistry: It is used as a building block for the synthesis of more complex molecules.
Biology: The compound is studied for its interactions with biological systems, particularly its antibacterial properties.
Medicine: It serves as a lead compound for the development of new antibacterial drugs.
Industry: The compound is used in the production of pharmaceuticals and other chemical products.
Comparison with Similar Compounds
Similar Compounds
Ciprofloxacin: A widely used fluoroquinolone antibiotic with a similar structure but different substituents.
Norfloxacin: Another fluoroquinolone with a similar core structure but different functional groups.
Ofloxacin: A fluoroquinolone with a similar mechanism of action but different pharmacokinetic properties.
Uniqueness
6-Fluoro-4-oxo-1,4-dihydroquinoline-3-carbonitrile is unique due to its specific functional groups, which confer distinct biological activities and chemical properties. The presence of the nitrile group at the 3rd position and the fluorine atom at the 6th position enhances its antibacterial activity and makes it a valuable compound for further research and development .
Properties
IUPAC Name |
6-fluoro-4-oxo-1H-quinoline-3-carbonitrile | |
---|---|---|
Details | Computed by LexiChem 2.6.6 (PubChem release 2019.06.18) | |
Source | PubChem | |
URL | https://pubchem.ncbi.nlm.nih.gov | |
Description | Data deposited in or computed by PubChem | |
InChI |
InChI=1S/C10H5FN2O/c11-7-1-2-9-8(3-7)10(14)6(4-12)5-13-9/h1-3,5H,(H,13,14) | |
Details | Computed by InChI 1.0.5 (PubChem release 2019.06.18) | |
Source | PubChem | |
URL | https://pubchem.ncbi.nlm.nih.gov | |
Description | Data deposited in or computed by PubChem | |
InChI Key |
BHNUHFWMNOYIAE-UHFFFAOYSA-N | |
Details | Computed by InChI 1.0.5 (PubChem release 2019.06.18) | |
Source | PubChem | |
URL | https://pubchem.ncbi.nlm.nih.gov | |
Description | Data deposited in or computed by PubChem | |
Canonical SMILES |
C1=CC2=C(C=C1F)C(=O)C(=CN2)C#N | |
Details | Computed by OEChem 2.1.5 (PubChem release 2019.06.18) | |
Source | PubChem | |
URL | https://pubchem.ncbi.nlm.nih.gov | |
Description | Data deposited in or computed by PubChem | |
Molecular Formula |
C10H5FN2O | |
Details | Computed by PubChem 2.1 (PubChem release 2019.06.18) | |
Source | PubChem | |
URL | https://pubchem.ncbi.nlm.nih.gov | |
Description | Data deposited in or computed by PubChem | |
Molecular Weight |
188.16 g/mol | |
Details | Computed by PubChem 2.1 (PubChem release 2021.05.07) | |
Source | PubChem | |
URL | https://pubchem.ncbi.nlm.nih.gov | |
Description | Data deposited in or computed by PubChem | |
Retrosynthesis Analysis
AI-Powered Synthesis Planning: Our tool employs the Template_relevance Pistachio, Template_relevance Bkms_metabolic, Template_relevance Pistachio_ringbreaker, Template_relevance Reaxys, Template_relevance Reaxys_biocatalysis model, leveraging a vast database of chemical reactions to predict feasible synthetic routes.
One-Step Synthesis Focus: Specifically designed for one-step synthesis, it provides concise and direct routes for your target compounds, streamlining the synthesis process.
Accurate Predictions: Utilizing the extensive PISTACHIO, BKMS_METABOLIC, PISTACHIO_RINGBREAKER, REAXYS, REAXYS_BIOCATALYSIS database, our tool offers high-accuracy predictions, reflecting the latest in chemical research and data.
Strategy Settings
Precursor scoring | Relevance Heuristic |
---|---|
Min. plausibility | 0.01 |
Model | Template_relevance |
Template Set | Pistachio/Bkms_metabolic/Pistachio_ringbreaker/Reaxys/Reaxys_biocatalysis |
Top-N result to add to graph | 6 |
Feasible Synthetic Routes
Disclaimer and Information on In-Vitro Research Products
Please be aware that all articles and product information presented on BenchChem are intended solely for informational purposes. The products available for purchase on BenchChem are specifically designed for in-vitro studies, which are conducted outside of living organisms. In-vitro studies, derived from the Latin term "in glass," involve experiments performed in controlled laboratory settings using cells or tissues. It is important to note that these products are not categorized as medicines or drugs, and they have not received approval from the FDA for the prevention, treatment, or cure of any medical condition, ailment, or disease. We must emphasize that any form of bodily introduction of these products into humans or animals is strictly prohibited by law. It is essential to adhere to these guidelines to ensure compliance with legal and ethical standards in research and experimentation.