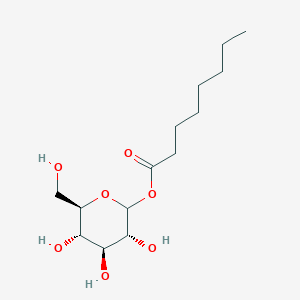
Octanoyl-D-glucopyranoside
Overview
Description
Octanoyl-D-glucopyranoside is a carbohydrate-based surfactant, specifically a glycolipid, which consists of a glucose molecule esterified with an octanoic acid. This compound is known for its amphiphilic properties, making it useful in various industrial and scientific applications. It is often considered a safe alternative to petroleum-based surfactants due to its renewable origin, high biodegradability, and low toxicity .
Mechanism of Action
Target of Action
Octanoyl-D-Glucopyranoside primarily targets the Probable cysteine desulfurase . This enzyme plays a crucial role in the removal of elemental sulfur and selenium atoms from L-cysteine, L-cystine, L-selenocysteine, and L-selenocystine to produce L-alanine .
Mode of Action
It is known to interact with its target, the probable cysteine desulfurase, and catalyze the removal of elemental sulfur and selenium atoms from certain amino acids . This interaction and the resulting changes contribute to its biological activity.
Biochemical Pathways
It is part of the class of organic compounds known as saccharolipids . These compounds have a structure where fatty acids are linked directly to a sugar backbone, forming structures that are compatible with membrane bilayers .
Pharmacokinetics
A related compound, piceid octanoate, has been reported to possess a better adme profile than resveratrol , suggesting that this compound might also have favorable pharmacokinetic properties.
Result of Action
It is known to be active against gram-positive bacteria, has bactericidal activity against both antibiotic-sensitive and resistant strains, and is likely to exert its primary antibacterial effect by damaging the cytoplasmic membrane .
Action Environment
The action of this compound can be influenced by various environmental factors. For instance, it has been shown that the compound can be synthesized in three non-aqueous reaction systems, namely organic solvents, ionic liquids, and co-solvent mixtures . This suggests that the compound’s action, efficacy, and stability can be influenced by the nature of the solvent environment.
Biochemical Analysis
Biochemical Properties
Octanoyl-D-glucopyranoside interacts with various enzymes, proteins, and other biomolecules. For instance, it has been found to be part of hybrid membranes composed of 1,2-dipalmitoyl-sn-glycero-3-phosphocholine (DPPC), cholesterol, and octyl-β-D-glucopyranoside (OGP) . These lipid/sugar materials could have potential properties to use as nanovesicles for drug delivery .
Cellular Effects
This compound has significant effects on various types of cells and cellular processes. It influences cell function by modifying thermodynamic parameters of membranes such as phase transition temperature, enthalpy change, and cooperativity . This implies changes in the fluidity and compactness of the membrane occurring at temperatures of biological relevance .
Molecular Mechanism
The molecular mechanism of this compound involves its interaction with cholesterol in the DPPC bilayer. The hydrophobic nature of certain substances, such as ibuprofen, leads to their incorporation into this hybrid core . This results in changes in the fluidity and compactness of the membrane .
Temporal Effects in Laboratory Settings
In laboratory settings, lipid vesicles containing this compound at 6.0 mM loaded with ibuprofen demonstrated good stability after 3 months of storage . This suggests that this compound has long-term effects on cellular function.
Transport and Distribution
This compound is likely transported and distributed within cells and tissues as part of lipid/sugar materials, which could be used as nanovesicles for drug delivery . Its interaction with cholesterol suggests it may be localized in areas where cholesterol is present .
Subcellular Localization
The subcellular localization of this compound is not fully known. Given its role in lipid/sugar materials that could be used as nanovesicles for drug delivery , it may be localized in areas of the cell involved in vesicle formation and transport.
Preparation Methods
Synthetic Routes and Reaction Conditions: The synthesis of Octanoyl-D-glucopyranoside typically involves the acylation of D-glucose with octanoic acid. One efficient method is the regioselective acylation of octyl β-D-glucopyranoside at low temperatures. This process involves treating the glucopyranoside with acyl halides to obtain the desired O-acylglucopyranosides at specific positions (C-2, C-3, and C-4) with high yields .
Industrial Production Methods: Industrial production of this compound can be achieved through enzymatic synthesis using engineered β-glucosidase in non-aqueous reaction systems. This method involves reverse hydrolysis reactions catalyzed by the enzyme in organic solvents and ionic liquids, resulting in high yields of the desired product .
Chemical Reactions Analysis
Types of Reactions: Octanoyl-D-glucopyranoside undergoes various chemical reactions, including:
Oxidation: The hydroxyl groups on the glucose moiety can be oxidized to form corresponding carbonyl compounds.
Reduction: The carbonyl groups can be reduced back to hydroxyl groups.
Substitution: The ester linkage can be hydrolyzed under acidic or basic conditions to yield the free acid and glucose.
Common Reagents and Conditions:
Oxidation: Common oxidizing agents include potassium permanganate and chromium trioxide.
Reduction: Reducing agents such as sodium borohydride and lithium aluminum hydride are used.
Substitution: Acidic or basic hydrolysis can be performed using hydrochloric acid or sodium hydroxide.
Major Products:
Oxidation: Formation of glucuronic acid derivatives.
Reduction: Regeneration of the original hydroxyl groups.
Substitution: Production of free octanoic acid and glucose.
Scientific Research Applications
Octanoyl-D-glucopyranoside has a wide range of applications in scientific research, including:
Chemistry: Used as a surfactant in various chemical reactions and processes.
Industry: Employed in the formulation of cleaning products, food, cosmetics, and drug carriers.
Comparison with Similar Compounds
Octyl β-D-glucopyranoside: Another glycolipid with similar surfactant properties.
Decyl β-D-glucopyranoside: A longer-chain analog with enhanced surfactant properties.
Phloretin 4’-O-(6’’-O-octanoyl)-α-D-glucopyranoside: Known for its inhibitory effects on enzymes like hexokinase.
Uniqueness: Octanoyl-D-glucopyranoside is unique due to its specific chain length and the balance between hydrophilic and lipophilic properties, making it highly effective as a surfactant and enzyme modulator. Its biodegradability and low toxicity further enhance its appeal for various applications .
Properties
IUPAC Name |
[(3R,4S,5S,6R)-3,4,5-trihydroxy-6-(hydroxymethyl)oxan-2-yl] octanoate | |
---|---|---|
Details | Computed by LexiChem 2.6.6 (PubChem release 2019.06.18) | |
Source | PubChem | |
URL | https://pubchem.ncbi.nlm.nih.gov | |
Description | Data deposited in or computed by PubChem | |
InChI |
InChI=1S/C14H26O7/c1-2-3-4-5-6-7-10(16)21-14-13(19)12(18)11(17)9(8-15)20-14/h9,11-15,17-19H,2-8H2,1H3/t9-,11-,12+,13-,14?/m1/s1 | |
Details | Computed by InChI 1.0.5 (PubChem release 2019.06.18) | |
Source | PubChem | |
URL | https://pubchem.ncbi.nlm.nih.gov | |
Description | Data deposited in or computed by PubChem | |
InChI Key |
MHQWMCFSLKPQTC-GQYPCLOQSA-N | |
Details | Computed by InChI 1.0.5 (PubChem release 2019.06.18) | |
Source | PubChem | |
URL | https://pubchem.ncbi.nlm.nih.gov | |
Description | Data deposited in or computed by PubChem | |
Canonical SMILES |
CCCCCCCC(=O)OC1C(C(C(C(O1)CO)O)O)O | |
Details | Computed by OEChem 2.1.5 (PubChem release 2019.06.18) | |
Source | PubChem | |
URL | https://pubchem.ncbi.nlm.nih.gov | |
Description | Data deposited in or computed by PubChem | |
Isomeric SMILES |
CCCCCCCC(=O)OC1[C@@H]([C@H]([C@@H]([C@H](O1)CO)O)O)O | |
Details | Computed by OEChem 2.1.5 (PubChem release 2019.06.18) | |
Source | PubChem | |
URL | https://pubchem.ncbi.nlm.nih.gov | |
Description | Data deposited in or computed by PubChem | |
Molecular Formula |
C14H26O7 | |
Details | Computed by PubChem 2.1 (PubChem release 2019.06.18) | |
Source | PubChem | |
URL | https://pubchem.ncbi.nlm.nih.gov | |
Description | Data deposited in or computed by PubChem | |
Molecular Weight |
306.35 g/mol | |
Details | Computed by PubChem 2.1 (PubChem release 2021.05.07) | |
Source | PubChem | |
URL | https://pubchem.ncbi.nlm.nih.gov | |
Description | Data deposited in or computed by PubChem | |
Retrosynthesis Analysis
AI-Powered Synthesis Planning: Our tool employs the Template_relevance Pistachio, Template_relevance Bkms_metabolic, Template_relevance Pistachio_ringbreaker, Template_relevance Reaxys, Template_relevance Reaxys_biocatalysis model, leveraging a vast database of chemical reactions to predict feasible synthetic routes.
One-Step Synthesis Focus: Specifically designed for one-step synthesis, it provides concise and direct routes for your target compounds, streamlining the synthesis process.
Accurate Predictions: Utilizing the extensive PISTACHIO, BKMS_METABOLIC, PISTACHIO_RINGBREAKER, REAXYS, REAXYS_BIOCATALYSIS database, our tool offers high-accuracy predictions, reflecting the latest in chemical research and data.
Strategy Settings
Precursor scoring | Relevance Heuristic |
---|---|
Min. plausibility | 0.01 |
Model | Template_relevance |
Template Set | Pistachio/Bkms_metabolic/Pistachio_ringbreaker/Reaxys/Reaxys_biocatalysis |
Top-N result to add to graph | 6 |
Feasible Synthetic Routes
Disclaimer and Information on In-Vitro Research Products
Please be aware that all articles and product information presented on BenchChem are intended solely for informational purposes. The products available for purchase on BenchChem are specifically designed for in-vitro studies, which are conducted outside of living organisms. In-vitro studies, derived from the Latin term "in glass," involve experiments performed in controlled laboratory settings using cells or tissues. It is important to note that these products are not categorized as medicines or drugs, and they have not received approval from the FDA for the prevention, treatment, or cure of any medical condition, ailment, or disease. We must emphasize that any form of bodily introduction of these products into humans or animals is strictly prohibited by law. It is essential to adhere to these guidelines to ensure compliance with legal and ethical standards in research and experimentation.