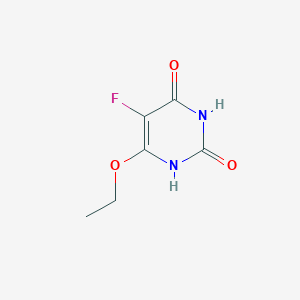
6-Ethoxy-5-fluorouracil
Overview
Description
6-Ethoxy-5-fluorouracil is a fluorinated derivative of uracil, a pyrimidine base found in RNAIt is structurally related to 5-fluorouracil, a well-known chemotherapeutic agent used in the treatment of various cancers .
Mechanism of Action
Target of Action
6-Ethoxy-5-fluorouracil, a derivative of 5-fluorouracil (5-FU), primarily targets the enzyme thymidylate synthase (TS) . TS plays a crucial role in DNA synthesis by converting deoxyuridylic acid to thymidylic acid . By inhibiting TS, this compound disrupts DNA synthesis, leading to cell death .
Mode of Action
The compound interacts with its target, TS, by forming a covalently bound ternary complex with the deoxyribonucleotide of the drug (FdUMP) and the folate cofactor, N5–10-methylenetetrahydrofolate . This interaction inhibits TS, thereby disrupting DNA synthesis and leading to cell death .
Biochemical Pathways
The inhibition of TS by this compound affects several biochemical pathways. It leads to the depletion of deoxythymidine triphosphate (dTTP), causing imbalances in the levels of other deoxynucleotides (dATP, dGTP, and dCTP) through various feedback mechanisms . This imbalance severely disrupts DNA synthesis and repair, resulting in lethal DNA damage . Additionally, TS inhibition results in the accumulation of dUMP, which might subsequently lead to increased levels of deoxyuridine triphosphate (dUTP) . Both dUTP and the 5-FU metabolite FdUTP can be misincorporated into DNA .
Pharmacokinetics
The pharmacokinetics of 5-FU, from which this compound is derived, is characterized by significant variability when dosing is based on body surface area . This variability suggests that individualized dosing strategies, potentially guided by pharmacokinetic monitoring, could improve therapeutic outcomes .
Result of Action
The primary result of this compound’s action is the disruption of DNA synthesis and repair, leading to cell death . This makes it an effective chemotherapeutic agent for a wide variety of neoplasms .
Action Environment
The action of this compound can be influenced by various environmental factors. For instance, the “mutual prodrug” approach has emerged as a potential tool to overcome undesirable physicochemical features and mitigate the side effects of approved drugs like 5-FU . This approach involves the pharmacologic manipulation of the intracellular pathways of a drug with another drug or natural compound, with the ultimate aim of either increasing the antitumor activity, decreasing the toxicity, or both .
Biochemical Analysis
Biochemical Properties
6-Ethoxy-5-fluorouracil, like its parent compound 5-FU, is likely to interact with various enzymes, proteins, and other biomolecules. 5-FU is known to be incorporated into RNA and DNA, leading to cytotoxicity and cell death . It is plausible that this compound may have similar interactions, although specific studies on this compound are needed to confirm this.
Cellular Effects
5-FU has been shown to cause cellular injury, influencing cell growth modulation, including loss or accumulation of S phase cells, G2/M block, and G1-S arrest . It’s possible that this compound may have similar effects on various types of cells and cellular processes.
Molecular Mechanism
The molecular mechanism of action of this compound is not well-defined. 5-FU exerts its effects at the molecular level through the misincorporation of fluoronucleotides into RNA and DNA and the inhibition of the nucleotide synthetic enzyme thymidylate synthase (TS) . It’s plausible that this compound may have a similar mechanism of action.
Temporal Effects in Laboratory Settings
Studies on 5-FU have shown that its effects can change over time, with potential impacts on cellular function .
Dosage Effects in Animal Models
Studies on 5-FU have shown that its reproductive toxicity often associates with developmental block, malformation, and ovarian damage in females, and alters the morphology of sexual organs, the levels of reproductive endocrine hormones, and the progression of spermatogenesis in males .
Metabolic Pathways
The metabolic pathways involving this compound are not well-defined. 5-FU is known to be metabolized in a three-step sequential metabolic pathway, with dihydropyrimidine dehydrogenase (DPD) being the initial and rate-limiting enzyme .
Transport and Distribution
Studies on 5-FU have shown that it can be transported and distributed within cells and tissues .
Subcellular Localization
The subcellular localization of this compound is not well-defined. Studies on 5-FU have shown that treatment with 5-FU leads to reconstruction of the bone marrow microenvironment, establishing an activated niche stimulating hematopoietic stem cells .
Preparation Methods
Synthetic Routes and Reaction Conditions
The synthesis of 6-Ethoxy-5-fluorouracil typically involves the fluorination of uracil derivatives. One common method includes the reaction of 5-fluorouracil with ethyl iodide in the presence of a base, such as potassium carbonate, to introduce the ethoxy group at the 6-position . The reaction is carried out under reflux conditions to ensure complete conversion.
Industrial Production Methods
Industrial production of this compound follows similar synthetic routes but on a larger scale. The process involves the use of high-purity reagents and optimized reaction conditions to maximize yield and purity. The product is then purified using techniques such as recrystallization or chromatography .
Chemical Reactions Analysis
Types of Reactions
6-Ethoxy-5-fluorouracil undergoes various chemical reactions, including:
Oxidation: The compound can be oxidized to form corresponding oxo derivatives.
Reduction: Reduction reactions can yield dihydro derivatives.
Substitution: Nucleophilic substitution reactions can occur at the fluorine or ethoxy positions.
Common Reagents and Conditions
Oxidation: Common oxidizing agents include potassium permanganate and hydrogen peroxide.
Reduction: Reducing agents such as sodium borohydride or lithium aluminum hydride are used.
Substitution: Nucleophiles like amines or thiols can be used under basic conditions.
Major Products Formed
The major products formed from these reactions include various substituted uracil derivatives, which can have different biological activities and properties .
Scientific Research Applications
6-Ethoxy-5-fluorouracil has several scientific research applications:
Chemistry: Used as a building block in the synthesis of more complex molecules.
Biology: Studied for its interactions with nucleic acids and enzymes.
Medicine: Investigated for its potential as an anticancer agent, similar to 5-fluorouracil.
Industry: Utilized in the development of new pharmaceuticals and agrochemicals.
Comparison with Similar Compounds
Similar Compounds
5-Fluorouracil: A widely used chemotherapeutic agent.
2-Ethoxy-5-fluoropyrimidin-4(1H)-one: Another fluorinated uracil derivative.
Uniqueness
6-Ethoxy-5-fluorouracil is unique due to its specific substitution pattern, which can influence its biological activity and pharmacokinetic properties. This makes it a valuable compound for developing new therapeutic agents with potentially improved efficacy and reduced side effects .
Properties
IUPAC Name |
6-ethoxy-5-fluoro-1H-pyrimidine-2,4-dione | |
---|---|---|
Details | Computed by LexiChem 2.6.6 (PubChem release 2019.06.18) | |
Source | PubChem | |
URL | https://pubchem.ncbi.nlm.nih.gov | |
Description | Data deposited in or computed by PubChem | |
InChI |
InChI=1S/C6H7FN2O3/c1-2-12-5-3(7)4(10)8-6(11)9-5/h2H2,1H3,(H2,8,9,10,11) | |
Details | Computed by InChI 1.0.5 (PubChem release 2019.06.18) | |
Source | PubChem | |
URL | https://pubchem.ncbi.nlm.nih.gov | |
Description | Data deposited in or computed by PubChem | |
InChI Key |
DHICDECWZLWEAR-UHFFFAOYSA-N | |
Details | Computed by InChI 1.0.5 (PubChem release 2019.06.18) | |
Source | PubChem | |
URL | https://pubchem.ncbi.nlm.nih.gov | |
Description | Data deposited in or computed by PubChem | |
Canonical SMILES |
CCOC1=C(C(=O)NC(=O)N1)F | |
Details | Computed by OEChem 2.1.5 (PubChem release 2019.06.18) | |
Source | PubChem | |
URL | https://pubchem.ncbi.nlm.nih.gov | |
Description | Data deposited in or computed by PubChem | |
Molecular Formula |
C6H7FN2O3 | |
Details | Computed by PubChem 2.1 (PubChem release 2019.06.18) | |
Source | PubChem | |
URL | https://pubchem.ncbi.nlm.nih.gov | |
Description | Data deposited in or computed by PubChem | |
Molecular Weight |
174.13 g/mol | |
Details | Computed by PubChem 2.1 (PubChem release 2021.05.07) | |
Source | PubChem | |
URL | https://pubchem.ncbi.nlm.nih.gov | |
Description | Data deposited in or computed by PubChem | |
Retrosynthesis Analysis
AI-Powered Synthesis Planning: Our tool employs the Template_relevance Pistachio, Template_relevance Bkms_metabolic, Template_relevance Pistachio_ringbreaker, Template_relevance Reaxys, Template_relevance Reaxys_biocatalysis model, leveraging a vast database of chemical reactions to predict feasible synthetic routes.
One-Step Synthesis Focus: Specifically designed for one-step synthesis, it provides concise and direct routes for your target compounds, streamlining the synthesis process.
Accurate Predictions: Utilizing the extensive PISTACHIO, BKMS_METABOLIC, PISTACHIO_RINGBREAKER, REAXYS, REAXYS_BIOCATALYSIS database, our tool offers high-accuracy predictions, reflecting the latest in chemical research and data.
Strategy Settings
Precursor scoring | Relevance Heuristic |
---|---|
Min. plausibility | 0.01 |
Model | Template_relevance |
Template Set | Pistachio/Bkms_metabolic/Pistachio_ringbreaker/Reaxys/Reaxys_biocatalysis |
Top-N result to add to graph | 6 |
Feasible Synthetic Routes
Disclaimer and Information on In-Vitro Research Products
Please be aware that all articles and product information presented on BenchChem are intended solely for informational purposes. The products available for purchase on BenchChem are specifically designed for in-vitro studies, which are conducted outside of living organisms. In-vitro studies, derived from the Latin term "in glass," involve experiments performed in controlled laboratory settings using cells or tissues. It is important to note that these products are not categorized as medicines or drugs, and they have not received approval from the FDA for the prevention, treatment, or cure of any medical condition, ailment, or disease. We must emphasize that any form of bodily introduction of these products into humans or animals is strictly prohibited by law. It is essential to adhere to these guidelines to ensure compliance with legal and ethical standards in research and experimentation.