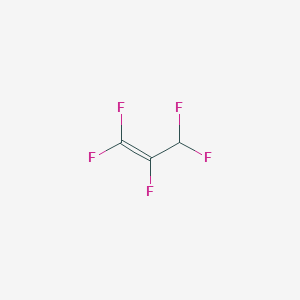
1,1,2,3,3-Pentafluoropropene
Overview
Description
1,1,2,3,3-Pentafluoropropene is an unsaturated fluorocarbon with the molecular formula C3HF5. It is a colorless gas with a molecular weight of 132.0321 .
Mechanism of Action
Target of Action
1,1,2,3,3-Pentafluoroprop-1-ene is an unsaturated fluorocarbon . It is primarily used as a precursor to hydrofluoroolefins (HFOs), which are used as refrigerants in air conditioners . Therefore, its primary targets are the components of air conditioning systems where it contributes to the cooling process.
Mode of Action
The compound interacts with its targets (air conditioning systems) by undergoing phase transitions. When the refrigerant evaporates, it absorbs heat from the surrounding environment, thereby cooling it. When it condenses, it releases the absorbed heat. The specific interactions of 1,1,2,3,3-Pentafluoroprop-1-ene with its targets are determined by its physical and chemical properties, including its boiling point and heat capacity .
Biochemical Pathways
Its role is primarily in the physical processes of heat transfer in air conditioning systems .
Pharmacokinetics
Its analogous properties in an industrial context would be its rate of evaporation and condensation, its distribution within an air conditioning system, and its stability under various conditions .
Result of Action
The primary result of the action of 1,1,2,3,3-Pentafluoroprop-1-ene is the cooling of an environment when used as a refrigerant in an air conditioning system. This is achieved through the absorption of heat during evaporation, followed by the release of heat during condensation .
Action Environment
The efficacy and stability of 1,1,2,3,3-Pentafluoroprop-1-ene as a refrigerant can be influenced by various environmental factors. These include the temperature and pressure of the environment, the specific design of the air conditioning system, and the presence of any contaminants. For example, its boiling point is -14.7±8.0 °C for the Z-isomer and -18.5 °C for the E-isomer , so its efficacy as a refrigerant would decrease in environments with temperatures below these points.
Preparation Methods
1,1,2,3,3-Pentafluoropropene can be synthesized through several methods. One common method involves the dehydrofluorination of 1,1,1,2,3,3-hexafluoropropane in the presence of hydrogen . Another method involves the reaction of 1,1,1,2,3,3-hexafluoropropane with trivalent chromium oxide or partly fluorinated trivalent chromium oxide in the gas state . These methods are cost-effective and yield high reactivity and selectivity.
Chemical Reactions Analysis
1,1,2,3,3-Pentafluoropropene undergoes various chemical reactions, including:
Oxidation: It can be oxidized to form products such as tetrafluoropropionaldehyde and trifluoropyruvaldehyde.
Reduction: The compound can be reduced using hydrogen in the presence of a hydrogenation catalyst.
Substitution: It can undergo substitution reactions with strong bases, releasing toxic gases.
Scientific Research Applications
1,1,2,3,3-Pentafluoropropene has several scientific research applications:
Chemistry: It is used as a monomer in the synthesis of fluorinated polymers. Its unique properties make it a valuable building block for generating molecules of higher value.
Biology and Medicine: The compound is under investigation for its potential use in clinical applications, such as a vapocoolant spray for numbing small boils before incision and drainage.
Comparison with Similar Compounds
1,1,2,3,3-Pentafluoropropene can be compared with other similar compounds such as:
1,1,1,3,3-Pentafluoropropane: This compound is used primarily for closed-cell spray foam insulation and has a high global warming potential.
1,2,3,3,3-Pentafluoropropene: Another isomer of pentafluoropropene, used as a precursor to HFOs.
2,3,3,3-Tetrafluoropropene: Used in the synthesis of fluorinated polymers and has been studied for its reactivity with rhodium(I) complexes.
Properties
IUPAC Name |
1,1,2,3,3-pentafluoroprop-1-ene | |
---|---|---|
Details | Computed by LexiChem 2.6.6 (PubChem release 2019.06.18) | |
Source | PubChem | |
URL | https://pubchem.ncbi.nlm.nih.gov | |
Description | Data deposited in or computed by PubChem | |
InChI |
InChI=1S/C3HF5/c4-1(2(5)6)3(7)8/h2H | |
Details | Computed by InChI 1.0.5 (PubChem release 2019.06.18) | |
Source | PubChem | |
URL | https://pubchem.ncbi.nlm.nih.gov | |
Description | Data deposited in or computed by PubChem | |
InChI Key |
NDMMKOCNFSTXRU-UHFFFAOYSA-N | |
Details | Computed by InChI 1.0.5 (PubChem release 2019.06.18) | |
Source | PubChem | |
URL | https://pubchem.ncbi.nlm.nih.gov | |
Description | Data deposited in or computed by PubChem | |
Canonical SMILES |
C(C(=C(F)F)F)(F)F | |
Details | Computed by OEChem 2.1.5 (PubChem release 2019.06.18) | |
Source | PubChem | |
URL | https://pubchem.ncbi.nlm.nih.gov | |
Description | Data deposited in or computed by PubChem | |
Molecular Formula |
C3HF5 | |
Details | Computed by PubChem 2.1 (PubChem release 2019.06.18) | |
Source | PubChem | |
URL | https://pubchem.ncbi.nlm.nih.gov | |
Description | Data deposited in or computed by PubChem | |
Molecular Weight |
132.03 g/mol | |
Details | Computed by PubChem 2.1 (PubChem release 2021.05.07) | |
Source | PubChem | |
URL | https://pubchem.ncbi.nlm.nih.gov | |
Description | Data deposited in or computed by PubChem | |
Retrosynthesis Analysis
AI-Powered Synthesis Planning: Our tool employs the Template_relevance Pistachio, Template_relevance Bkms_metabolic, Template_relevance Pistachio_ringbreaker, Template_relevance Reaxys, Template_relevance Reaxys_biocatalysis model, leveraging a vast database of chemical reactions to predict feasible synthetic routes.
One-Step Synthesis Focus: Specifically designed for one-step synthesis, it provides concise and direct routes for your target compounds, streamlining the synthesis process.
Accurate Predictions: Utilizing the extensive PISTACHIO, BKMS_METABOLIC, PISTACHIO_RINGBREAKER, REAXYS, REAXYS_BIOCATALYSIS database, our tool offers high-accuracy predictions, reflecting the latest in chemical research and data.
Strategy Settings
Precursor scoring | Relevance Heuristic |
---|---|
Min. plausibility | 0.01 |
Model | Template_relevance |
Template Set | Pistachio/Bkms_metabolic/Pistachio_ringbreaker/Reaxys/Reaxys_biocatalysis |
Top-N result to add to graph | 6 |
Feasible Synthetic Routes
Disclaimer and Information on In-Vitro Research Products
Please be aware that all articles and product information presented on BenchChem are intended solely for informational purposes. The products available for purchase on BenchChem are specifically designed for in-vitro studies, which are conducted outside of living organisms. In-vitro studies, derived from the Latin term "in glass," involve experiments performed in controlled laboratory settings using cells or tissues. It is important to note that these products are not categorized as medicines or drugs, and they have not received approval from the FDA for the prevention, treatment, or cure of any medical condition, ailment, or disease. We must emphasize that any form of bodily introduction of these products into humans or animals is strictly prohibited by law. It is essential to adhere to these guidelines to ensure compliance with legal and ethical standards in research and experimentation.