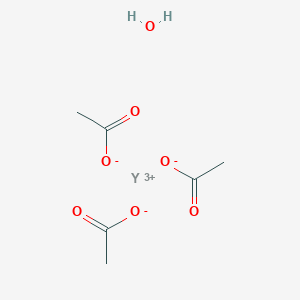
Yttrium(III) acetate hydrate
Overview
Description
Yttrium(III) acetate hydrate is a chemical compound with the formula (CH₃CO₂)₃Y·xH₂O. It is a white, crystalline substance that is moderately soluble in water and decomposes to yttrium oxide upon heating . Yttrium is a rare earth element, and its compounds are known for their various applications in materials science, electronics, and catalysis.
Mechanism of Action
Target of Action
Yttrium(III) acetate hydrate is a compound that primarily targets the synthesis of various yttrium compounds, including yttrium oxides and yttrium fluorides . It is also used as a catalyst for ethylene polymerization .
Mode of Action
The mode of action of this compound involves its interaction with other compounds during the synthesis process. For instance, in the synthesis of sodium yttrium fluoride (NaYF4) nanoparticles, yttrium acetate is mixed with oleic acid in octadecene and heated to form Y(oleate)3 . This compound is then reacted with ammonium fluoride and sodium hydroxide in methanol at modest temperatures to form NaYF4 nanoparticles .
Biochemical Pathways
The biochemical pathways affected by this compound are primarily related to the synthesis of yttrium compounds. The compound’s solubility and low thermal decomposition temperature make it attractive for hydrothermal reactions and co-precipitation processing before calcination .
Pharmacokinetics
Its solubility in water and mineral acids suggests that it could be readily absorbed and distributed in an aqueous environment.
Result of Action
The result of this compound’s action is the formation of various yttrium compounds, including yttrium oxides and yttrium fluorides . These compounds have various applications, such as in the production of synthetic garnets and in the creation of nanoparticles that can convert two photons of near-infrared (NIR) light into visible light .
Action Environment
The action of this compound can be influenced by environmental factors. For instance, its solubility can be affected by the presence of water and mineral acids . Additionally, its reactivity can be influenced by temperature, as it is used in reactions that involve heating .
Biochemical Analysis
Biochemical Properties
Yttrium(III) acetate hydrate plays a significant role in biochemical reactions, particularly as a catalyst. It interacts with various enzymes, proteins, and other biomolecules, facilitating or inhibiting specific biochemical processes. For instance, this compound can act as a catalyst in the polymerization of ethylene, interacting with enzymes involved in the polymerization process Additionally, it can form complexes with proteins, altering their structure and function
Cellular Effects
This compound has been shown to influence various cellular processes and functions. It can affect cell signaling pathways, gene expression, and cellular metabolism. For example, this compound can modulate the activity of certain signaling pathways, such as the MAPK/ERK pathway, which is involved in cell proliferation and differentiation . Additionally, it can influence gene expression by binding to specific transcription factors or DNA sequences, thereby regulating the transcription of target genes. These effects on cellular processes highlight the potential of this compound in therapeutic applications and biomedical research.
Molecular Mechanism
The molecular mechanism of this compound involves its interactions with biomolecules at the molecular level. It can bind to enzymes, inhibiting or activating their activity. For instance, this compound can inhibit the activity of certain proteases by binding to their active sites, preventing substrate binding and catalysis Additionally, it can interact with DNA and RNA, affecting their stability and function
Temporal Effects in Laboratory Settings
In laboratory settings, the effects of this compound can change over time due to its stability and degradation. The compound is generally stable at room temperature but can degrade under certain conditions, such as high temperatures or exposure to light . Over time, the degradation of this compound can lead to changes in its biochemical properties and effects on cellular function. Long-term studies in vitro and in vivo have shown that the compound can have lasting effects on cellular processes, including alterations in gene expression and cellular metabolism.
Dosage Effects in Animal Models
The effects of this compound can vary with different dosages in animal models. At low doses, the compound may have minimal effects on cellular function and overall health. At higher doses, this compound can exhibit toxic or adverse effects, such as hepatotoxicity and nephrotoxicity . Studies have shown that the compound can accumulate in specific organs, such as the liver and kidneys, leading to tissue damage and impaired function. Understanding the dosage effects of this compound is crucial for its safe and effective use in biomedical applications.
Metabolic Pathways
This compound is involved in various metabolic pathways, interacting with enzymes and cofactors that regulate metabolic processes. For example, the compound can influence the activity of enzymes involved in the citric acid cycle, affecting metabolic flux and metabolite levels Additionally, this compound can interact with cofactors such as NAD+ and FAD, altering their redox states and impacting cellular metabolism
Transport and Distribution
The transport and distribution of this compound within cells and tissues are critical for its biochemical effects. The compound can be transported across cell membranes via specific transporters or binding proteins . Once inside the cell, this compound can localize to specific cellular compartments, such as the cytoplasm or nucleus, where it exerts its effects on cellular processes. The distribution of the compound within tissues can also vary, with higher concentrations observed in specific organs, such as the liver and kidneys . Understanding the transport and distribution of this compound is crucial for its effective use in biomedical applications.
Subcellular Localization
The subcellular localization of this compound is essential for its activity and function. The compound can be directed to specific cellular compartments or organelles through targeting signals or post-translational modifications For example, this compound can localize to the mitochondria, where it can influence mitochondrial function and energy metabolism Additionally, the compound can be found in the nucleus, where it can interact with DNA and transcription factors, affecting gene expression
Preparation Methods
Synthetic Routes and Reaction Conditions: Yttrium(III) acetate hydrate can be synthesized by reacting yttrium oxide or yttrium hydroxide with acetic acid. The reaction typically involves dissolving yttrium oxide in acetic acid, followed by crystallization to obtain the hydrate form. The general reaction is as follows:
Y2O3+6CH3COOH→2(CH3CO2)3Y+3H2O
The reaction is usually carried out at room temperature, and the product is obtained by evaporating the solvent and allowing the crystals to form .
Industrial Production Methods: Industrial production of this compound follows similar principles but on a larger scale. The process involves the controlled reaction of yttrium oxide with acetic acid in large reactors, followed by crystallization and purification steps to ensure high purity of the final product .
Chemical Reactions Analysis
Types of Reactions: Yttrium(III) acetate hydrate undergoes various chemical reactions, including:
Thermal Decomposition: Upon heating, it decomposes to form yttrium oxide.
Complex Formation: It can form complexes with various ligands, such as polyaminocarboxylates.
Common Reagents and Conditions:
Oxidation: this compound can be oxidized to form yttrium oxide.
Reduction: It can be reduced under specific conditions to form lower oxidation state compounds.
Substitution: The acetate groups can be substituted with other ligands in the presence of suitable reagents.
Major Products:
Yttrium Oxide: Formed during thermal decomposition.
Complexes: Formed with various ligands during complexation reactions.
Scientific Research Applications
Yttrium(III) acetate hydrate has numerous applications in scientific research:
Comparison with Similar Compounds
Ytterbium(III) acetate hydrate: Similar in structure and used in similar applications.
Erbium(III) acetate hydrate: Another rare earth acetate with comparable properties.
Yttrium(III) nitrate hexahydrate: Used in similar industrial and research applications.
Uniqueness: Yttrium(III) acetate hydrate is unique due to its specific thermal decomposition behavior and its ability to form stable complexes with a wide range of ligands. This makes it particularly useful in applications requiring high thermal stability and complexation capabilities .
Properties
IUPAC Name |
yttrium(3+);triacetate;hydrate | |
---|---|---|
Details | Computed by LexiChem 2.6.6 (PubChem release 2019.06.18) | |
Source | PubChem | |
URL | https://pubchem.ncbi.nlm.nih.gov | |
Description | Data deposited in or computed by PubChem | |
InChI |
InChI=1S/3C2H4O2.H2O.Y/c3*1-2(3)4;;/h3*1H3,(H,3,4);1H2;/q;;;;+3/p-3 | |
Details | Computed by InChI 1.0.5 (PubChem release 2019.06.18) | |
Source | PubChem | |
URL | https://pubchem.ncbi.nlm.nih.gov | |
Description | Data deposited in or computed by PubChem | |
InChI Key |
JRKVGRAQLBXGQB-UHFFFAOYSA-K | |
Details | Computed by InChI 1.0.5 (PubChem release 2019.06.18) | |
Source | PubChem | |
URL | https://pubchem.ncbi.nlm.nih.gov | |
Description | Data deposited in or computed by PubChem | |
Canonical SMILES |
CC(=O)[O-].CC(=O)[O-].CC(=O)[O-].O.[Y+3] | |
Details | Computed by OEChem 2.1.5 (PubChem release 2019.06.18) | |
Source | PubChem | |
URL | https://pubchem.ncbi.nlm.nih.gov | |
Description | Data deposited in or computed by PubChem | |
Molecular Formula |
C6H11O7Y | |
Details | Computed by PubChem 2.1 (PubChem release 2019.06.18) | |
Source | PubChem | |
URL | https://pubchem.ncbi.nlm.nih.gov | |
Description | Data deposited in or computed by PubChem | |
Molecular Weight |
284.05 g/mol | |
Details | Computed by PubChem 2.1 (PubChem release 2021.05.07) | |
Source | PubChem | |
URL | https://pubchem.ncbi.nlm.nih.gov | |
Description | Data deposited in or computed by PubChem | |
Retrosynthesis Analysis
AI-Powered Synthesis Planning: Our tool employs the Template_relevance Pistachio, Template_relevance Bkms_metabolic, Template_relevance Pistachio_ringbreaker, Template_relevance Reaxys, Template_relevance Reaxys_biocatalysis model, leveraging a vast database of chemical reactions to predict feasible synthetic routes.
One-Step Synthesis Focus: Specifically designed for one-step synthesis, it provides concise and direct routes for your target compounds, streamlining the synthesis process.
Accurate Predictions: Utilizing the extensive PISTACHIO, BKMS_METABOLIC, PISTACHIO_RINGBREAKER, REAXYS, REAXYS_BIOCATALYSIS database, our tool offers high-accuracy predictions, reflecting the latest in chemical research and data.
Strategy Settings
Precursor scoring | Relevance Heuristic |
---|---|
Min. plausibility | 0.01 |
Model | Template_relevance |
Template Set | Pistachio/Bkms_metabolic/Pistachio_ringbreaker/Reaxys/Reaxys_biocatalysis |
Top-N result to add to graph | 6 |
Feasible Synthetic Routes
Disclaimer and Information on In-Vitro Research Products
Please be aware that all articles and product information presented on BenchChem are intended solely for informational purposes. The products available for purchase on BenchChem are specifically designed for in-vitro studies, which are conducted outside of living organisms. In-vitro studies, derived from the Latin term "in glass," involve experiments performed in controlled laboratory settings using cells or tissues. It is important to note that these products are not categorized as medicines or drugs, and they have not received approval from the FDA for the prevention, treatment, or cure of any medical condition, ailment, or disease. We must emphasize that any form of bodily introduction of these products into humans or animals is strictly prohibited by law. It is essential to adhere to these guidelines to ensure compliance with legal and ethical standards in research and experimentation.