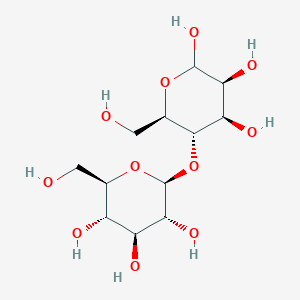
beta-D-glucosyl-(1->4)-D-mannopyranose
Overview
Description
β-D-Glucosyl-(1→4)-D-mannopyranose is a disaccharide composed of a β-linked D-glucose residue at the reducing end and a D-mannopyranose residue connected via a β(1→4) glycosidic bond. This structure is a fundamental unit of glucomannans, heteropolysaccharides found in plant cell walls and certain microbial extracellular matrices . Glucomannans consist of alternating β(1→4)-linked D-mannose and D-glucose residues, contributing to their role in structural integrity and water retention . The β(1→4) linkage confers resistance to enzymatic hydrolysis compared to α-linked analogs, making it a critical determinant of biodegradability and functional interactions with enzymes like β-mannosidases .
Preparation Methods
Enzymatic Synthesis Methods
Enzymatic synthesis offers high stereochemical fidelity and avoids the need for extensive protecting group strategies. Two primary enzymatic pathways have been explored: phosphorylase-catalyzed synthesis and glycosyltransferase-mediated approaches.
Phosphorylase-Catalyzed Synthesis
Phosphorylases catalyze reversible phosphorolysis of glycosidic bonds, enabling the synthesis of disaccharides from sugar 1-phosphates and acceptors. The enzyme BT1033 from Bacteroides thetaiotaomicron has been identified as a key catalyst for β-1,4-mannosyl linkages. In the presence of α-D-glucose 1-phosphate (Glc1P) and D-mannose, BT1033 facilitates the formation of β-D-glucosyl-(1→4)-D-mannopyranose with a kcat/Km of 1.2 × 103 M−1s−1 for mannose, demonstrating efficient catalytic turnover.
A related phosphorylase from Cellvibrio gilvus has been employed to synthesize analogous disaccharides. Using 1,5-anhydro-D-fructose (AF) as an acceptor and Glc1P as a donor, this enzyme produced β-D-glucosyl-(1→4)-AF at 30°C and pH 7.0, though the product exhibited instability with a half-life of 17 hours under these conditions. This instability underscores the need for rapid purification in enzymatic workflows.
Table 1: Kinetic Parameters of Phosphorylases in β-1,4-Disaccharide Synthesis
Enzyme Source | Substrate Pair | kcat (s−1) | Km (mM) | kcat/Km (M−1s−1) |
---|---|---|---|---|
B. thetaiotaomicron | Glc1P + Mannose | 4.8 ± 0.3 | 4.0 ± 0.5 | 1.2 × 103 |
C. gilvus | Glc1P + AF | 0.15 ± 0.02 | 2.5 ± 0.3 | 60 |
Glycosyltransferase-Mediated Approaches
Glycosyltransferases (GTs) represent another enzymatic avenue, utilizing nucleotide sugar donors (e.g., UDP-glucose) to transfer monosaccharides to acceptors. While GTs offer regiospecificity, their dependence on costly nucleotide donors limits industrial scalability. Recent advances in ATP recycling systems and immobilized enzyme technologies have improved cost-effectiveness, though yields for β-D-glucosyl-(1→4)-D-mannopyranose remain unreported in public literature.
Chemical Synthesis Strategies
Chemical synthesis provides greater control over protecting group strategies and glycosylation conditions but requires meticulous optimization to avoid side reactions.
Protecting Group Strategies
Selective protection of hydroxyl groups is critical for directing glycosylation. Benzyl (Bn) and acetyl (Ac) groups are commonly used. For example, 2,3,4,6-tetra-O-benzyl-D-mannopyranose serves as a key intermediate, with the C2 hydroxyl left unprotected to participate in glycosylation.
Glycosylation Reactions
Glycosylation of protected mannopyranose with glucosyl donors (e.g., trichloroacetimidates) under Lewis acid catalysis (e.g., BF3·OEt2) achieves β-selectivity. A flow chemistry approach using a tandem Wittig–Michael reaction at 180°C and 130°C in sequential reactors yielded trans-2-(C-glycosyl)acetates with 60% efficiency, though epimerization at C2 was observed under basic conditions.
Table 2: Optimization of Glycosylation Conditions in Flow Chemistry
Reactor 1 Temp (°C) | Reactor 2 Temp (°C) | Residence Time (min) | Yield (%) | α:β Ratio |
---|---|---|---|---|
200 | 145 | 65 | 43 | 0.5:1 |
180 | 130 | 45 | 60 | 0.5:1 |
Comparative Analysis of Methodologies
Enzymatic methods excel in stereoselectivity and mild reaction conditions but face challenges in product stability and enzyme availability. Chemical synthesis, while scalable, struggles with side reactions and requires toxic reagents. Industrial production currently favors enzymatic routes due to lower environmental impact, though hybrid approaches combining enzymatic glycosylation with chemical protecting groups are emerging.
Industrial-Scale Production Considerations
Large-scale synthesis necessitates cost-effective substrates and robust purification. Microbial fermentation using engineered E. coli strains expressing BT1033 phosphorylase has been proposed, leveraging glucose and mannose as feedstocks. However, downstream processing must address the compound’s propensity for β-elimination, which generates ascopyrone T and glucose under neutral pH. Stabilization strategies, such as lyophilization at acidic pH or encapsulation, are under investigation.
Chemical Reactions Analysis
Types of Reactions: Beta-D-glucosyl-(1->4)-D-mannopyranose can undergo various chemical reactions, including:
Oxidation: The hydroxyl groups can be oxidized to form aldehydes or carboxylic acids.
Reduction: The carbonyl group in the glucose or mannose unit can be reduced to form alcohols.
Substitution: Hydroxyl groups can be substituted with other functional groups, such as halogens or alkyl groups.
Common Reagents and Conditions:
Oxidation: Reagents like periodic acid or bromine water are commonly used.
Reduction: Sodium borohydride or lithium aluminum hydride are typical reducing agents.
Substitution: Reagents like thionyl chloride or alkyl halides are used for substitution reactions.
Major Products:
Oxidation: Aldonic acids or uronic acids.
Reduction: Sugar alcohols like sorbitol or mannitol.
Substitution: Halogenated sugars or alkylated sugars.
Scientific Research Applications
Beta-D-glucosyl-(1->4)-D-mannopyranose has a wide range of applications in scientific research:
Chemistry: Used as a building block for the synthesis of more complex carbohydrates and glycoconjugates.
Biology: Studied for its role in cell signaling and recognition processes.
Medicine: Investigated for its potential as a prebiotic, promoting the growth of beneficial gut bacteria.
Industry: Utilized in the production of biofuels, food additives, and pharmaceuticals.
Mechanism of Action
The mechanism of action of beta-D-glucosyl-(1->4)-D-mannopyranose involves its interaction with specific enzymes and receptors in biological systems. It can act as a substrate for glycosidases, which hydrolyze the glycosidic bond to release glucose and mannose. These monosaccharides can then enter metabolic pathways, providing energy and building blocks for cellular processes.
Comparison with Similar Compounds
Structural and Functional Comparisons
The table below summarizes key structural analogs and their properties:
Key Differentiators
Linkage Configuration: β(1→4) linkages (e.g., in β-D-glucosyl-mannopyranose) are hydrolyzed by β-specific enzymes (e.g., β-mannosidases) , whereas α-linked analogs (e.g., 2-O-α-D-mannopyranosyl-mannose) are substrates for α-mannosidases . Lactose (β(1→4) Gal-Glc) shares a similar linkage but differs in biological context, serving as a nutrient rather than a structural component .
Biological Roles: Glucomannans contribute to plant cell wall flexibility and dietary fiber functions , while synthetic α-linked mannose derivatives (e.g., methyl α-D-Glcp-(1→2)-β-D-Manp) are engineered to stimulate immune responses .
Enzymatic Susceptibility: The β(1→4) glucosyl-mannose bond resists mammalian digestive enzymes, unlike lactose, which is efficiently cleaved by lactase .
Physicochemical Properties
- Solubility: β-D-glucosyl-(1→4)-mannopyranose is highly water-soluble due to the hydrophilic nature of glucose and mannose units, whereas β(1→4)-linked mannobiose exhibits lower solubility due to increased hydrogen bonding between mannose residues .
- Thermal Stability : β-linked disaccharides generally exhibit higher thermal stability than α-linked analogs, as seen in glucomannans’ resistance to gelatinization .
Biological Activity
Overview
Beta-D-glucosyl-(1->4)-D-mannopyranose is a disaccharide composed of a beta-D-glucose molecule linked to a D-mannose molecule via a 1->4 glycosidic bond. This compound is recognized for its significant biological activities, particularly in the fields of nutrition, microbiology, and biochemistry. Its potential applications as a prebiotic and its role in various metabolic pathways make it a compound of interest for researchers.
- Chemical Formula : C₁₂H₂₂O₁₁
- CAS Number : 27452-49-9
- Molecular Weight : 342.31 g/mol
Biological Functions
1. Prebiotic Effects
Beta-D-glucosyl-(1->4)-D-mannopyranose has been studied for its prebiotic properties, promoting the growth of beneficial gut bacteria such as Bifidobacterium and Lactobacillus. These bacteria play crucial roles in maintaining gut health and enhancing immune function. Research indicates that this disaccharide can stimulate the proliferation of these beneficial microorganisms, thereby contributing to improved gut flora composition and overall health .
2. Metabolic Pathways
The metabolism of beta-D-glucosyl-(1->4)-D-mannopyranose involves enzymatic reactions facilitated by specific glycoside hydrolases. For instance, enzymes like BT1033 from Bacteroides thetaiotaomicron have been identified to catalyze the reversible phosphorolysis of related compounds, indicating a sophisticated metabolic pathway that utilizes this disaccharide efficiently .
Case Studies
Case Study 1: Prebiotic Potential
A study conducted on the effects of beta-D-glucosyl-(1->4)-D-mannopyranose on gut microbiota demonstrated that supplementation led to a significant increase in populations of beneficial bacteria compared to control groups. This was measured using real-time PCR techniques to quantify bacterial DNA levels before and after supplementation .
Case Study 2: Enzymatic Activity
Research highlighted the role of specific enzymes in the breakdown and utilization of beta-D-glucosyl-(1->4)-D-mannopyranose. The enzyme BT1033 was shown to enhance the efficiency of energy extraction from this disaccharide, presenting an alternative pathway for ATP generation compared to traditional methods involving ATP-dependent hexokinases .
Comparative Analysis with Similar Compounds
Compound Name | Structure Type | Biological Activity |
---|---|---|
Beta-D-galactosyl-(1->4)-D-glucosyl-(1<->1)-N-acylsphingosine | Glycolipid | Cell signaling and immune modulation |
Beta-D-glucosyl-(1->4)-beta-D-galactopyranose | Disaccharide | Prebiotic effects and gut health improvement |
Beta-D-glucosyl-(1->4)-beta-D-glucopyranose | Disaccharide | Energy source and metabolic substrate |
Q & A
Basic Research Questions
Q. How can β-D-glucosyl-(1→4)-D-mannopyranose be detected and quantified in polysaccharide mixtures?
- Methodological Answer : The phenol-sulfuric acid colorimetric assay is a robust method for detecting reducing sugars, including oligosaccharides like β-D-glucosyl-(1→4)-D-mannopyranose. The assay involves reacting the sugar with phenol and concentrated sulfuric acid, producing an orange-yellow color measurable at 490 nm. For complex mixtures, coupling this with paper partition chromatography or HPLC improves specificity .
Table 1: Common Synthetic Intermediates for β-D-Glucosyl-(1→4)-D-Mannopyranose Derivatives
Table 2: Key Analytical Parameters for β-D-Glucosyl-(1→4)-D-Mannopyranose
Technique | Key Signals/Parameters | Application Example |
---|---|---|
-NMR | δ 100–105 ppm (anomeric carbons) | Distinguishing α/β configurations |
MALDI-TOF MS | m/z 349.1 [M+Na] | Confirming molecular weight |
FT-IR | 3400 cm (O-H), 1070 cm (C-O-C) | Detecting glycosidic linkages |
Properties
IUPAC Name |
(2R,3S,4S,5R,6S)-2-(hydroxymethyl)-6-[(2R,3S,4R,5S)-4,5,6-trihydroxy-2-(hydroxymethyl)oxan-3-yl]oxyoxane-3,4,5-triol | |
---|---|---|
Details | Computed by LexiChem 2.6.6 (PubChem release 2019.06.18) | |
Source | PubChem | |
URL | https://pubchem.ncbi.nlm.nih.gov | |
Description | Data deposited in or computed by PubChem | |
InChI |
InChI=1S/C12H22O11/c13-1-3-5(15)6(16)9(19)12(22-3)23-10-4(2-14)21-11(20)8(18)7(10)17/h3-20H,1-2H2/t3-,4-,5-,6+,7-,8+,9-,10-,11?,12+/m1/s1 | |
Details | Computed by InChI 1.0.5 (PubChem release 2019.06.18) | |
Source | PubChem | |
URL | https://pubchem.ncbi.nlm.nih.gov | |
Description | Data deposited in or computed by PubChem | |
InChI Key |
GUBGYTABKSRVRQ-LNCRCTFVSA-N | |
Details | Computed by InChI 1.0.5 (PubChem release 2019.06.18) | |
Source | PubChem | |
URL | https://pubchem.ncbi.nlm.nih.gov | |
Description | Data deposited in or computed by PubChem | |
Canonical SMILES |
C(C1C(C(C(C(O1)OC2C(OC(C(C2O)O)O)CO)O)O)O)O | |
Details | Computed by OEChem 2.1.5 (PubChem release 2019.06.18) | |
Source | PubChem | |
URL | https://pubchem.ncbi.nlm.nih.gov | |
Description | Data deposited in or computed by PubChem | |
Isomeric SMILES |
C([C@@H]1[C@H]([C@@H]([C@H]([C@@H](O1)O[C@@H]2[C@H](OC([C@H]([C@H]2O)O)O)CO)O)O)O)O | |
Details | Computed by OEChem 2.1.5 (PubChem release 2019.06.18) | |
Source | PubChem | |
URL | https://pubchem.ncbi.nlm.nih.gov | |
Description | Data deposited in or computed by PubChem | |
Molecular Formula |
C12H22O11 | |
Details | Computed by PubChem 2.1 (PubChem release 2019.06.18) | |
Source | PubChem | |
URL | https://pubchem.ncbi.nlm.nih.gov | |
Description | Data deposited in or computed by PubChem | |
Molecular Weight |
342.30 g/mol | |
Details | Computed by PubChem 2.1 (PubChem release 2021.05.07) | |
Source | PubChem | |
URL | https://pubchem.ncbi.nlm.nih.gov | |
Description | Data deposited in or computed by PubChem | |
Disclaimer and Information on In-Vitro Research Products
Please be aware that all articles and product information presented on BenchChem are intended solely for informational purposes. The products available for purchase on BenchChem are specifically designed for in-vitro studies, which are conducted outside of living organisms. In-vitro studies, derived from the Latin term "in glass," involve experiments performed in controlled laboratory settings using cells or tissues. It is important to note that these products are not categorized as medicines or drugs, and they have not received approval from the FDA for the prevention, treatment, or cure of any medical condition, ailment, or disease. We must emphasize that any form of bodily introduction of these products into humans or animals is strictly prohibited by law. It is essential to adhere to these guidelines to ensure compliance with legal and ethical standards in research and experimentation.